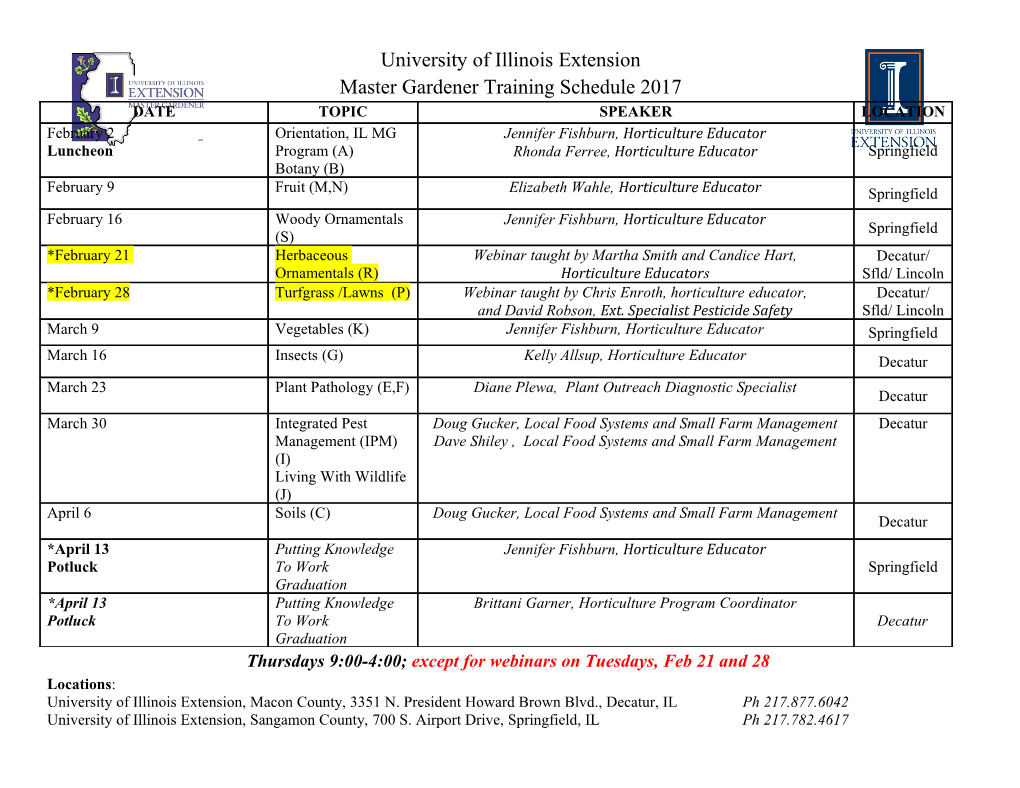
University of Nebraska - Lincoln DigitalCommons@University of Nebraska - Lincoln Faculty Publications in the Biological Sciences Papers in the Biological Sciences 2006 Microorganisms pumping iron: Anaerobic microbial iron oxidation and reduction Karrie A. Weber University of Nebraska-Lincoln, [email protected] Laurie A. Achenbach Southern Illinois University Carbondale, [email protected] John D. Coates University of California, Berkeley, [email protected] Follow this and additional works at: https://digitalcommons.unl.edu/bioscifacpub Part of the Life Sciences Commons Weber, Karrie A.; Achenbach, Laurie A.; and Coates, John D., "Microorganisms pumping iron: Anaerobic microbial iron oxidation and reduction" (2006). Faculty Publications in the Biological Sciences. 204. https://digitalcommons.unl.edu/bioscifacpub/204 This Article is brought to you for free and open access by the Papers in the Biological Sciences at DigitalCommons@University of Nebraska - Lincoln. It has been accepted for inclusion in Faculty Publications in the Biological Sciences by an authorized administrator of DigitalCommons@University of Nebraska - Lincoln. Published in Nature Reviews Microbiology 4 (October 2006), pp. 752-764; doi: 10.1038/nrmicro1490 Copyright © 2006 Nature Publishing Group. Used by permission. Microorganisms pumping iron: Anaerobic microbial iron oxidation and reduction Karrie A. Weber,1 Laurie A. Achenbach,2 & John D. Coates 1 1. Department of Plant and Microbial Biology, 271 Koshland Hall, University of California, Berkeley, Berkeley, California, 94720 USA. 2. Department of Microbiology, Southern Illinois University, Carbondale, Illinois, 62901 USA. Corresponding author — John D. Coates, email [email protected] Abstract Iron (Fe) has long been a recognized physiological requirement for life, yet for many microorganisms that persist in water, soils and sediments, its role extends well beyond that of a nutritional necessity. Fe(II) can function as an elec- tron source for iron-oxidizing microorganisms under both oxic and anoxic conditions and Fe(III) can function as a terminal electron acceptor under anoxic conditions for iron-reducing microorganisms. Given that iron is the fourth most abundant element in the Earth’s crust, iron redox reactions have the potential to support substantial micro- bial populations in soil and sedimentary environments. As such, biological iron apportionment has been described as one of the most ancient forms of microbial metabolism on Earth, and as a conceivable extraterrestrial metabo- lism on other iron-mineral-rich planets such as Mars. Furthermore, the metabolic versatility of the microorganisms involved in these reactions has resulted in the development of biotechnological applications to remediate contami- nated environments and harvest energy. At pH values at or above a circumneutral pH (~pH both the Archaea and Bacteria domains are capable 7), iron (Fe) exists primarily as insoluble, solid- of metabolically exploiting the favourable redox po- phase minerals in the divalent ferrous (Fe(II)) or tri- tential between the Fe(III)/Fe(II) couple and vari- valent ferric (Fe(III)) oxidation states1. The solubil- ous electron donors or acceptors. In this way, Fe(II) is ity of Fe(III) increases with decreasing pH values2. used as an electron donor to provide reducing equiv- Decreasing pH values also enhance the stability of alents for the assimilation of carbon into biomass by Fe(II), and below pH 4.0, Fe(II) primarily exists as lithotrophic FOM under both oxic and anoxic condi- an aqueous species, even in the presence of oxygen. tions, and Fe(III) can be used as a terminal electron The biogeochemical role of Fe(II)-oxidizing micro- acceptor under anaerobic conditions for lithotrophic organisms (FOM) in acidic environments has been and heterotrophic Fe(III)-reducing microorganisms well established (reviewed in Reference 3). At a cir- (FRM) (Figure 1). This Review discusses the intricate cumneutral pH, microbial iron redox cycling can biogeochemical role of microbially mediated iron ox- significantly affect the geochemistry of hydromor- idation and reduction in suboxic environments, de- phic soils (that is, soils showing poor drainage) and tailing the metabolisms, the microorganisms and the Lithotrophic sediments, leading to the degradation of organic mechanisms described so far. A lithotrophic matter, mineral dissolution and weathering, the for- organism uses mation of geologically significant minerals, and the Iron cycling: the microbial redox workout an inorganic mobilization or immobilization of various anions In the anoxic zone of neoteric environments at pH substrate and cations, including contaminants.1, 4, 5 >4.0, Fe(III) oxides are readily reduced and pro- (usually of The redox transition between the Fe(II) and vide an important electron sink for both chemical mineral origin) Fe(III) valence states has a fundamental role in mod- and biological processes. It is now established that to obtain energy ern environmental biogeochemistry and was prob- microbial reduction of Fe(III) oxide minerals by for growth. ably an important biogeochemical process on early FRM primarily controls the Fe(III)-reductive pro- Heterotrophic Earth. Before microbially mediated iron redox re- cess in non-sulphidogenic sedimentary environ- 6 A heterotrophic actions were discovered, abiotic mechanisms were ments (Figure 1). Even in some sulphidogenic en- organism thought to dominate environmental iron redox vironments (for example, some marine sediments) requires organic chemistry. However, it is now accepted that micro- where Fe(III) reduction is traditionally thought to compounds as a bial metabolism primarily controls iron redox chem- result from an abiotic reaction with biogenic hydro- carbon source. istry in most environments. Microorganisms from gen sulphide (H2S), direct enzymatic Fe(III) reduc- 752 A N A EROBIC MICROBI A L IRON OXID ATION A ND REDUCTION 753 tion by FRM has been shown to be substantial, and the activity of these organisms might account for as much as 90% of the oxidation of organic matter7. Organic carbon compounds are not the only elec- tron donors that FRM are capable of utilizing. These microorganisms are also capable of utilizing inor- Suboxic ganic electron donors such as hydrogen (H2) (re- viewed in Reference 6). Ammonium might have a An environment role in Fe(III) reduction as an electron donor8, how- with a partial ever, direct pure-culture evidence is still required pressure of oxygen that is to support this observation. The activity of FRM re- substantially sults in the generation of aqueous Fe(II) (Fe(II)aq), lower than the and solid-phase Fe(II)-bearing minerals (Fe(II)s) in- atmospheric cluding siderite, vivianite and geologically signifi- oxygen content. cant mixed-valence Fe(II)–Fe(III) minerals, such as magnetite and green rust.9, 10 The ubiquity and phy- Anoxic logenetic diversity of FRM, combined with the el- An environment emental abundance of iron in the earth’s crust, es- lacking oxygen. tablishes the global significance of this metabolic process.6 Figure 1. The microbially mediated iron redox cycle. Neoteric environments Similarly, microbially mediated Fe(II) oxidation Microorganisms have a significant role mediating iron ox- idation and reduction reactions in soils and sedimentary Modern is also known to contribute to the dynamic iron bio- environments. geochemical cycle at circumneutral pH in both oxic environments. The reduction of Fe(III) oxides occurs in the absence of oxygen. The re-oxidation of the biogenic Fe(II) and anoxic environments (Figure 1). The recent can occur through several biological mechanisms and is Electron sink identification of FOM in various aquatic and sedi- not simply limited to abiotic reactions with molecular oxy- A compound mentary systems — both freshwater and marine — gen. The regeneration of Fe(III) in the anoxic environment that receives indicates that Fe(II) undergoes both biotic and abi- promotes a dynamic iron redox cycle. electrons as otic oxidation in the environment. Abiotic oxidation an endpoint of an oxidative of Fe(II)aq can be mediated by reaction with oxidized manganese (Mn(IV)) species or by the diffusion of reaction. Fe(II)aq into an oxic environment where it subse- Fe(III) oxides produced through the activity of these Bioturbation quently reacts with molecular oxygen (O2). Disrup- organisms potentially function as terminal electron The disturbance tion of sediments by macrophytes and macrofauna acceptors for FRM, thereby perpetuating a dynamic of sediment can induce particle mixing and aeration, result- microbially mediated iron redox cycle (Figure 1). layers by ing in the subsequent oxidation of both Fe(II)aq and biological Fe(II)s (References 11, 12). However, so far, microbi- Microbial Fe(II) oxidation activity. ally mediated oxidative processes in direct associa- The microbial oxidation of Fe(II) coupled to the re- tion with bioturbation have not been studied to any duction of oxygen in environments at acidic and Microaerophilic significant extent, with such studies being limited circumneutral pH values has been recognized for An organism to the rhizosphere.13, 14, 15 Microaerophilic FOM ca- more than a century (References 16, 28 and refer- that is an obligate pable of competing with the abiotic oxidation kinet- ences therein). Yet, despite this historical knowl- anaerobe but ics between oxygen and Fe(II) have been shown to edge, the geological significance of aerobic Fe(II) ox- can survive in contribute to iron cycling
Details
-
File Typepdf
-
Upload Time-
-
Content LanguagesEnglish
-
Upload UserAnonymous/Not logged-in
-
File Pages14 Page
-
File Size-