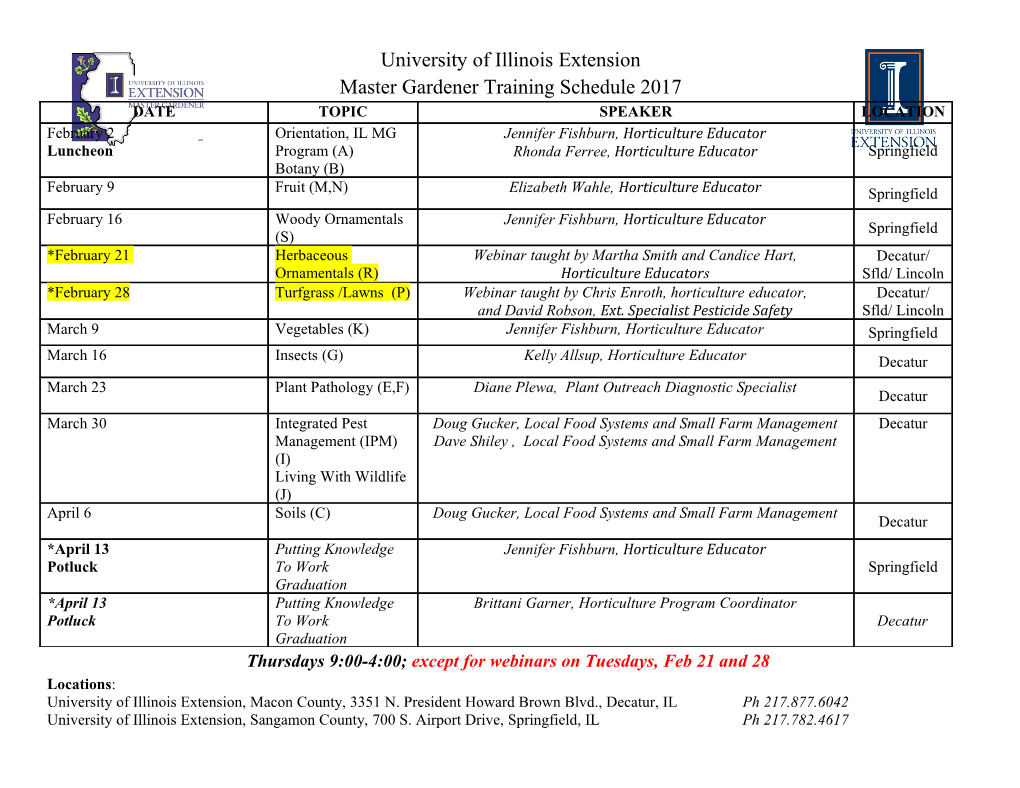
VITREOUS ENAMEL A HIGHLY EFFECTIVE MATERIAL COMPOUND J.C. Wendel, Wendel Email, Dillenburg, Germany P. Hellmold, Univ. Clausthal-Zellerfeld, Germany Abstract Mixed alkali effect Vitreous enamel is presented to be a highly effective material compound with designable properties. In general One would expect a direct line between ionic an enamel is a special glass, with a thermal expansion rate conductivity and glass stoichiometry and would suggest precisely adapted to it’s substrate. It’ s a bulk solid that variations in conductivity are related to the presence quenched from the melt – exhibiting a glass transition of certain chemical entities in glass. temperature. This is a general difference to amorphous materials deposited by sputtering or evaporation. This paper concentrates on the electrical properties and the adherence on the substrate. ELECTRICAL PROPERTIES Most of the glasses are electrical insulators. The specific electrical resistance of insulating enamels at room temperature is approximately 10 12 Ωcm, which decreases to approximately 10 5 Ωcm at 400°C. Of course the isolating properties of the enamel depend of the thickness of the enamel layer. Especially for the dielectric strength this matters. The existence of semi conducting glasses is known since about forty years. They are used for photovoltaic application and xerography. These glasses show an electronic conductivity. It has been made good progress in understanding the mechanisms of ion and electron transport in glasses. Electronic conductivity is achieved when elements with more than one oxidation level (Fe, Mn in silicate boron- glasses, V in phosphate- glasses) are built in the glass [1]. In this case we find also Figure 1: Conductivity and Molar volume in the system a dependence from the oxygen partial pressure of the (Li 2O/K 2O)*2SiO 2 at 150°C [3]. smelt. But mixing of alkalis in enamel produces a pronounced minimum (4 orders of magnitude) in the conductivity. The effect is illustrated in the figure 1 for potassium- Effect of composition elements lithium-disilicate (conductivity vs. mole fraction). The Nernst-Einstein equation is giving a good Physical properties like the molar volume do show the description for the electrical conductivity: The diffusion expected additive relationship. rate is mainly determining the effect [2]. • Charge transport is mostly done by cations. • Alkali metal ions have the highest diffusion rates. • A glass free of network modifiers is having a low conductivity. • BaO, SrO, PbO, CaO and SiO 2 decrease the conductivity due to the effect of polarisation and the blocking of channels. • Na 2O, Li 2O, K 2O, Rb 2O and Cs 2O increase the conductivity approximately in the order of their appearance in the list (Na 2O most). • The mixing of alkali oxides has a considerable effect. Figure 2: Specific electric resistance by substitution of Cs 2O in the glass 0,15 (Cs,M) 2O*0,85 SiO 2 [4]. The effect is universal to all ion conducting glasses. Mechanistic model Each type of alkali modifies the network structure to its The cation mobility and the immobility of the anions own requirements : Li +-ions create for themselves smaller can be intuitively understood by Figure 4. The Warren sites than do K + ions. Therefore there can be no rapid Biscoe version of the structure of an alkali silicate glass “exchange of sites” between different alkali. Figure 2 reduced to two dimensions shows the cations placed in shows the same effect for the specific resistance for a “holes” inside the glass structure. Caesium glass replacing Caesium by other ions. The substitution of Cs 2O in the glass 0,15 (Cs,M) 2O*0,85 SiO 2 by different alkali oxides leads to a maximum for the electric resistance at a molar fraction of approximately 0,5 [4]. The effect of temperature In general the specific resistance decreases as the temperature increases, while the dielectric constant increases. The increase of the temperature doesn’t have much influence on the polarisability of the ions, but the mobility of the ions increases very much which has an influence on the dielectric constant too [5]. Figure 4: A schematic representation of the structure of an alkali silicate glass showing the possibilities of cationic motion [7]. The possibilities of localized and extended cationic motion within an anionic silicate framework is shown. The shape of the channels inside the silicate framework is largely predetermined by the partially broken (and usually modified) network. Frequency dependence and Dissipation factor The dielectric constant also depends from the applied frequency. This is easy to understand, as the cations may Figure 3: Temperature dependence of the specific electric only follow the applied electric field at lower frequencies. resistance [6]. Therefore the dielectric constant decreases with increasing frequency. The amount of energy consumed e.g. for the motion of the ions to build up an electrical Figure 3 shows the temperature dependence of the current corresponds to tan δ - the dissipation factor. Just specific electric resistance of different enamels: a PbO as the dielectric constant also the dissipation factor containing enamel free of alkali oxides (a), a conventional depends from the composition of the glass, temperature white line enamel (b), an enamel with a higher content of and frequency. Figure 5 is a schematic depiction of the alkali oxide (c) and an antimony enamel (d) [6]. We find theoretical addition of the different dissipation typical Arrhenius plots showing the extrinsic conductivity contributions [8]: Curve 1 describes the conduction due to vibration of the network and opening of channels losses. With decreasing temperature and increasing for the migration of the ions. In the case of water frequency the possibilities for the motion of the cations adsorption on the enamel surface a special surface becomes lower and the conduction losses decrease. Curve conductivity may be found due to the formation of 2 describes the relaxation losses: the ions may follow the hydroxyl ions. A higher conductivity on the surface may applied field only with jumps over a small potential be also due to the enrichment of alkali ions on the surface barrier – these losses appear mostly at low frequencies. At resulting from the process of smelting the enamel onto the higher frequencies may appear a resonance with the substrate. natural oscillation of the ions. Big ions (curve 3) have a slower natural oscillation therefore the resonance appears iron surface and an electrolytic corrosion which is mostly at lower frequencies. Finally the network may start to caused by a reduction of adhesive oxides (CoO or NiO) oscillate in larger areas, the result is a deformation which [10]. Figure 7 shows the thermodynamics for the most leads to the deformation losses (curve 4) which are probable reactions which lead to metallic Cobalt – the expected mainly at lower temperatures. The full line reactions for NiO are similar. The reactions are calculated results from the addition of all contributions. for wüstite (Fe 1-xO). The corresponding chemical reaction are pointed out simplified (FeO). It is obvious that the most probable reaction is the reduction by intermediate formed wüstite [11]. Figure 5: Schematic depiction of the variation of the dissipation factor with the frequency [8]. This theoretical approach doesn’t have a scale on the Figure 7: Free reaction enthalpies as a function of the axis of tan δ. To find a scale we compare with figure 6. temperature for CoO [11]. The presence of adhesive oxides is increasing very much the surface roughness of the iron. Dietzel explained the way of acting of the adhesive oxides and the surface roughening. Sometimes we find small particles of iron made by disproportion of wustite to magnetite and iron. These particles we find usually at the border iron/enamel. The cobalt film on the surface has a thickness of approx. 0,01 µm. The cathodic areas (without cobalt) are attacked selectively and so cavities are created. Figure 8 shows a schematic depiction of the galvanic process during enamelling of iron. Figure 6: The influence of the chemical composition on the dissipation factor at a frequency of 1 kHz [9]. At low frequencies the mobility of the alkali ions is significant. With a decreasing content of alkali or an increase of boron oxide or calcium oxide in the Na 2O*5SiO 2 Glass the dissipation factor is decreasing [9]. The increase due to aluminium oxide is explained by the expansion of the network forming larger cycle structures which support the alkali ions with larger channels to move [5]. Figure 8: Schematic depiction of the galvanic process ADHERENCE ON IRON SUBSTRATES [12]. A main point for the use of enamelled products is the On the other hand a good adhesive iron oxide layer is adherence of the enamel on it’s substrate. The enamel needed [13]. The main phases should be magnetite which does not stick like a film on the surface, instead of that a increases the adherence, hematite decreases the chemical reaction between the substrate and the enamel adherence. The thickness of the intermediate oxide layer occurs. So the adherence on iron substrates may be is important [11]. Figure 9 shows the phase diagram (first explained on the one hand by mechanical interlocking of kind) of the system Fe – Fe 2O3. The areas for the stability the materials. Important factors are the roughness of the of the three important iron oxides are shown. DEVELOPMENT OF NEW ENAMELS When a new enamel is designed various factors must be respected: The viscosity and the surface tension of the enamel must be corresponding to the working conditions of the enameller. The dilatation must be adapted to the substrate. The adherence must be moderated by a ground coat or adapted for a direct on enamel. The surface and the elasticity must be to the needs of the final user, just as the question if the enamel must be smooth, glossy or matt.
Details
-
File Typepdf
-
Upload Time-
-
Content LanguagesEnglish
-
Upload UserAnonymous/Not logged-in
-
File Pages4 Page
-
File Size-