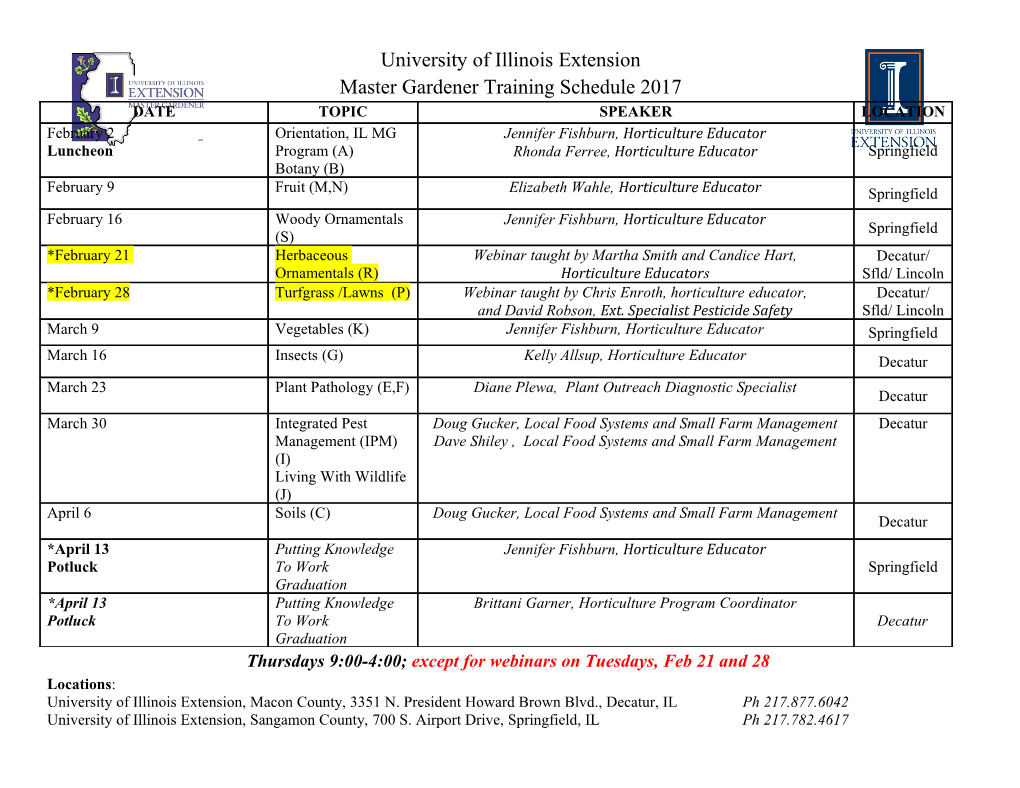
CDNA CLONING AND CHARACTERIZATION OF ENZYMES THAT SYNTHESIZE BILE ACIDS, VITAMIN D AND WAXES APPROVED BY SUPERVISORY COMMITTEE David Russell, Ph.D. Helen Hobbs, M.D. Elliott Ross, Ph.D. James Waddle, Ph.D. CDNA CLONING AND CHARACTERIZATION OF ENZYMES THAT SYNTHESIZE BILE ACIDS, VITAMIN D AND WAXES by JEFFREY BINYAN CHENG DISSERTATION / THESIS Presented to the Faculty of the Graduate School of Biomedical Sciences The University of Texas Southwestern Medical Center at Dallas In Partial Fulfillment of the Requirements For the Degree of DOCTOR OF PHILOSOPHY The University of Texas Southwestern Medical Center at Dallas Dallas, Texas June, 2006 Copyright by Jeffrey Binyan Cheng, 2006 All Rights Reserved cDNA CLONING AND CHARACTERIZATION OF ENZYMES THAT SYNTHESIZE BILE ACIDS, VITAMIN D AND WAXES Publication No. Jeffrey Binyan Cheng, Ph.D. The University of Texas Southwestern Medical Center at Dallas, 2006 Supervising Professor: David Russell, Ph.D. Countless enzymes are required for the synthesis of the diverse array of lipids found in nature. The identification and characterization of five different lipid metabolizing 5 enzymes are reported here. The 3β-hydroxy-∆ -C27-steroid oxidoreductase (C27 3β-HSD) enzyme catalyzes a step in bile acid synthesis. Subjects with mutations in the encoding gene fail to synthesize bile acids and develop liver disease. Fifteen patients were screened and twelve different mutations were identified in the C27 3β-HSD gene. Vitamin D is required for normal bone metabolism and maintenance of serum calcium levels. The conversion of vitamin D into an active ligand requires 25-hydroxylation. I report here the identification by expression cloning of a cytochrome P450 (CYP2R1) with iv vitamin D 25-hydroxylase activity. A patient with low circulating levels of 25- hydroxyvitamin D and classic symptoms of vitamin D deficiency was identified. Molecular analysis of this individual revealed homozygosity for a transition mutation in the CYP2R1 gene causing the substitution of a proline for a leucine in the protein and eliminating vitamin D 25-hydroxylase enzyme activity. These data identify CYP2R1 as a biologically relevant vitamin D 25-hydroxylase and reveal the molecular basis of a human genetic disease, selective 25-hydroxyvitamin D deficiency. The reduction of fatty acids to fatty alcohols, by a fatty acyl-CoA reductase enzyme, is required for the synthesis of wax monoesters and ether lipids. Using a bioinformatics approach, the first two mammalian fatty acyl-CoA reductase genes (FAR1 and FAR2) were identified. The two mouse FAR enzymes, which share 57% sequence identity at the amino acid level, have differing substrate specificities and tissue distributions implying unique physiological roles for each. Wax monoesters are synthesized by the esterification of fatty alcohols and fatty acids. A mammalian enzyme that catalyzes this reaction has not been isolated. Here, I report the identification by expression cloning of a wax synthase gene. Co-expression of cDNAs specifying FAR1 and wax synthase led to the synthesis of wax monoesters. The data suggests that wax monoester synthesis in mammals involves a two step biosynthetic pathway catalyzed by fatty acyl-CoA reductase and wax synthase enzymes. v TABLE OF CONTENTS Abstract iv Table of Contents vi Publications viii List of Figures and Tables ix List of Abbreviations xii Chapter 1 Introduction 1 5 Chapter 2 Molecular genetics of 3β-hydroxy-∆ -C27-steroid oxidoreductase deficiency in 16 patients with loss of bile acid synthesis and liver disease A. Introduction 33 B. Materials and Methods 36 C. Results 40 D. Discussion 44 E. Figures and Tables 49 Chapter 3 De-orphanization of cytochrome P450 2R1: a microsomal vitamin D 25- hydroxylase A. Introduction 67 B. Materials and Methods 70 C. Results 78 D. Discussion 88 E. Figures 94 Chapter 4 Genetic evidence that the human CYP2R1 enzyme is a key vitamin D 25- hydroxylase A. Introduction 112 B. Materials and Methods 114 C. Results 118 D. Discussion 122 E. Figures 127 vi Chapter 5 Identification of two mammalian fatty acyl-coenzyme A reductases with different substrate specificities and tissue distributions A. Introduction 137 B. Materials and Methods 139 C. Results 149 D. Discussion 156 E. Figures 161 Chapter 6 Expression cloning of mammalian wax synthase cDNAs encoding a member of the diacylglycerol acyltransferase family A. Introduction 175 B. Materials and Methods 178 C. Results 189 D. Discussion 196 E. Figures 201 Bibliography 217 vii PUBLICATIONS Cheng, J. B., Jacquemin, E., Gerhard, M., Nazer, H., Cresteil, D., Heubi, J.E., Setchell, K.D., and Russell, D. W. (2004). Molecular genetics of 3beta-hydroxy-Delta5-C27-steroid oxidoreductase deficiency in 16 patients with loss of bile acid synthesis and liver disease. J Clin Endocrinol Metab 88, 1,833-1,841. Cheng, J. B., Motola, D. L., Mangelsdorf, D. J., and Russell, D. W. (2003). De- orphanization of cytochrome P450 2R1, a microsomal vitamin D 25-hydroxylase. J Biol Chem 278, 38,084-38,093. Cheng, J. B., Levine, M.A., Bell, N.H., Mangelsdorf, D.J., and Russell, D. W. (2004). Genetic evidence that the human CYP2R1 enzyme is a key vitamin D 25-hydroxylase. Proc Natl Acad Sci USA 101, 7,711-7,715. Cheng, J. B. and Russell, D. W. (2004). Mammalian wax biosynthesis: I. Identification of two fatty acyl-Coenzyme A reductases with different substrate specificities and tissue distributions. J Biol Chem 279, 37,789-37,797. Cheng, J. B. and Russell, D. W. (2004). Mammalian wax biosynthesis: II. Expression cloning of wax synthase cDNAs encoding a member of the acyltransferase enzyme family. J Biol Chem 279, 37,798-37,807. viii LIST OF FIGURES AND TABLES Chapter 1 Figure 1 Biochemical steps involved in conversion of cholesterol to bile Acids Figure 2 Two different forms of vitamin D Figure 3 Steps involved in endogenous synthesis of vitamin D Figure 4 Activation and inactivation pathways of vitamin D Figure 5 The biochemical steps of mammalian wax biosynthesis Figure 6 Subclasses of phospholipids Chapter 2 Table 1 Summary of clinical data from 16 patients with C27 3β-HSD deficiency Table 2 Molecular genetics of C27 3β-HSD deficiency Figure 1 Reaction catalyzed by the C27 3β-HSD enzyme Figure 2 Pedigrees of two families with C27 3β-HSD deficiency Figure 3 DNA sequence analyses of mutations in the HSD3B7 gene Figure 4 Structure of the HSD3B7 gene and locations of mutations identified in subjects with neonatal cholestasis Figure 5 RNA blotting analysis of HSD3B7 mutations Figure 6 Effects of HSD3B7 mutations on C27 3β-HSD enzyme activity Chapter 3 Figure 1 Expression cloning of vitamin D 25-hydroxylase cDNA Figure 2 Amino acid sequences and gene structure of cytochrome P450 2R1 Figure 3 Activation of vitamin D3 by CYP2R1 Figure 4 Activation of vitamin D3 by cytochrome P450 enzymes ix Figure 5 Response of VDR/VDRE-Luciferase reporter system to P450 expression vectors and vitamin D3 Figure 6 Activation of vitamin D3 and vitamin D2 by microsomal versus mitochondrial P450 enzymes Figure 7 Inactivation of vitamin D metabolites by expression of CYP24A1 vitamin D 24-hydroxylase Figure 8 Biochemical and immunological identification of CYP2R1 vitamin D metabolites Figure 9 Tissue distributions of mouse vitamin D hydroxylase mRNAs Chapter 4 Figure 1 Structure of the human CYP2R1 gene Figure 2 DNA sequence analysis of mutation in CYP2R1 vitamin D 25-hydroxylase Figure 3 Biochemical assay of CYP2R1 vitamin D 25-hydroxylase enzyme activity Figure 4 L99P mutation in CYP2R1 causes loss of vitamin D3 activation Figure 5 Response of normal and L99P CYP2R1 enzymes to increasing concentrations of vitamin D3 and vitamin D2 Chapter 5 Figure 1 Amino acid sequences of mouse, plant and insect fatty acyl-CoA reductase (FAR) enzymes Figure 2 Tissue distributions of mouse FAR1 and FAR2 mRNAs Figure 3 Expression of mouse and human FAR enzymes in HEK 293 cells Figure 4 Substrate and cofactor preferences of mouse FAR1 enzyme Figure 5 Substrate preferences of mouse FAR1 and FAR2 enzymes Figure 6 Subcellular localization of mouse FAR1 and FAR2 enzymes x Chapter 6 Figure 1 Expression cloning of wax synthase cDNAs from mouse preputial gland and expression mouse and human wax synthase enzyme activities in HEK 293 cells Figure 2 Comparison of mouse and human wax synthases Figure 3 Substrate preferences of the mouse wax synthase enzyme Figure 4 Wax synthase activity of acyltransferase family members Figure 5 Acyltransferase activity of wax synthase enzyme. Figure 6 Subcellular localization of mouse wax synthase enzyme Figure 7 Tissue distributions of mouse wax synthase mRNA Figure 8 Reconstitution of mammalian wax biosynthetic pathway in cell lysates of transfected HEK 293 cells xi LIST OF ABBREVIATIONS 25-hydroxycholesterol [3H]cholest-5-ene-3ß,25-diol 7-dehydrocholesterol 5,7,-cholestadien-3β-ol ACAT acyl-CoA:cholesterol acyltransferase ACC acetyl-CoA carboxylase Adx adrenodoxin BSA bovine serum albumin 5 C27 3ß-HSD 3ß-hydroxy- -C27-steroid oxidoreductase CHAPS 3-[(3-cholamidopropyl)dimethylammonio]-1- propanesulfonic acid CMV cytomegalovirus CoA acyl-coenzyme A CT threshold value CYP cytochrome P450 CYP39A1 24-hydroxycholesterol 7α-hydroxylase CYP46A1 cholesterol 24-hydroxylase CYP7A1 cholesterol 7α hydroxylase CYP7B1 oxysterol 7α-hydroxylase DGAT acyl-CoA:diacylglycerol acyltransferase DHAP dihydroxyacetone phosphate DHAPAT dihydroxyacetone phosphate acyltransferase xii DMEM Dulbecco's modified Eagle's medium E. coli Escherichia coli FAR fatty acyl-coenzyme A reductase FAS fatty acid synthase FXR farnesoid X receptor
Details
-
File Typepdf
-
Upload Time-
-
Content LanguagesEnglish
-
Upload UserAnonymous/Not logged-in
-
File Pages257 Page
-
File Size-