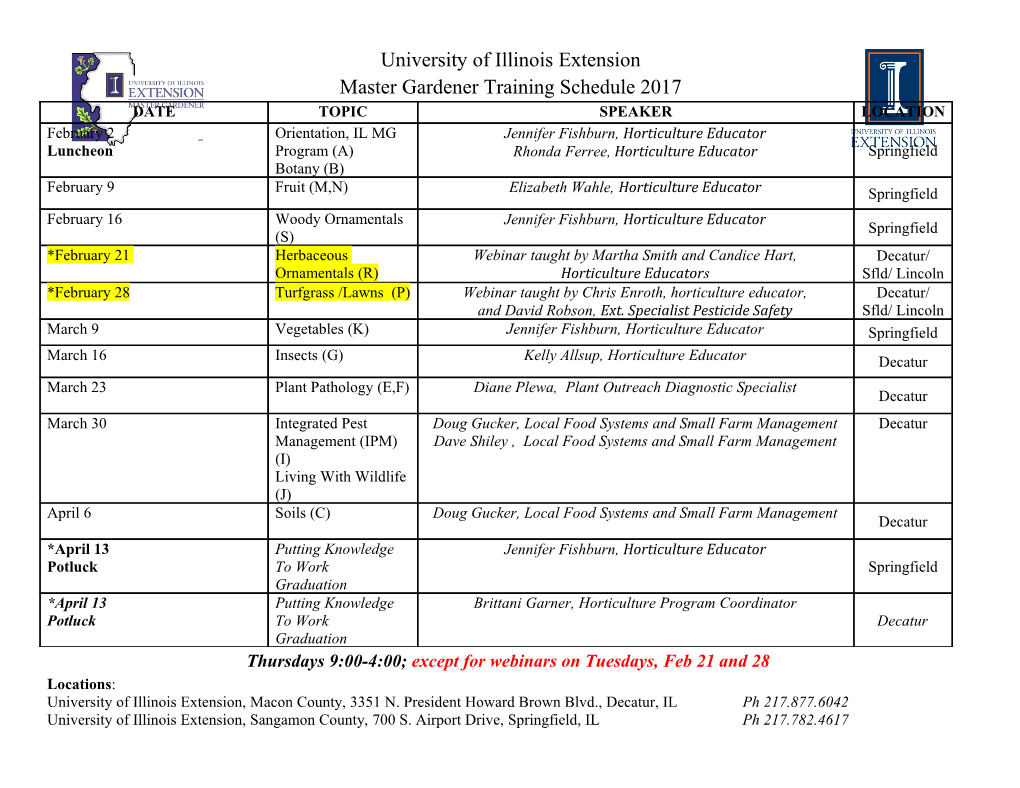
NUMERICAL SIMULATION OF AN ENGLISH EQUESTRIAN SADDLETREE Abdul-Hakeem .O. Noibi (MSc BSc) A thesis submitted in partial fulfilment of the requirements of the University of Wolverhampton for the degree of Master of Philosophy October 2011 This work or any part thereof has not previously been presented in any form to the University or to any other body whether for the purposes of assessment, publication or for any other purpose (unless otherwise indicated). Save for any express acknowledgments, references and/or bibliographies cited in the work, I confirm that the intellectual content of the work is the result of my own efforts and of no other person. The right of Abdul-Hakeem .O. Noibi to be identified as author of this work is asserted in accordance with ss. 77 and 78 of the Copyright, Designs and Patents Act 1988. At this date copyright is owned by the author. Signature ……………………………………… Date …………………………………………... Dedicated to the memory of my parents ii ABSTRACT The manufacture of horse riding saddlery in the UK is centred in Walsall in the West Midlands. The local industry faces stiff competition from cheap imports in local markets and dwindling exports to international markets. To continue to provide affordable high end products, which the local industry is reputed for, it is essential to develop an understanding of the functional and performance requirements of the saddletree based on scientific and engineering methods thereby laying a foundation for a methodical approach to product development. Through the measurement of surface data from a physical artefact, a 3 dimensional CAD geometrical replica of the English jumping saddletree was developed. Assumptions for the loading and boundary conditions identical to the user environment were obtained from available literature on the movements of the horseback, parameters for a jumping horse, saddletree test data, and, interface contact measurements acquired using a pressure mat. In addition, material properties data were obtained from available literature. Nonlinear static numerical models were subsequently developed and parametric studies were performed to determine the relationship between the deformation of the saddletree and the bending loads. Furthermore, nonlinear transient dynamic numerical models were developed and parametric studies performed to determine the response of the pommel to impact loads. The models were found to be sensitive to loading, material, and geometric iii parameters. From cantilever tests, the stiffness of the saddletree was found to be between 3.63 N/mm and 4.68 N/mm, and the steel reinforcement plates increased the stiffness by a factor of up to 2.3 times. Simply supported, the stiffness of the saddletree was between 526.62 N/mm and 596.16 N/mm, and the steel reinforcement plates increased the stiffness by a factor of up to 5.5 times. In addition, the simply supported models were sensitive to the wood laminate stacking sequence. Furthermore, the dynamic models showed that the steel reinforcement plates dampened the oscillations in the pommel after impact with a rigid body at 7 m/s, 8.5 m/s, and 10 m/s. The numerical cantilever models were validated with experimental data while interface pressure mat measurements validated interface contact stresses between the deformable bodies and the rigid body surfaces. Interface pressure mat results exhibited pressure hot spots and uneven load distributions underneath the saddletree. Peak and average pressures were 82.7 KPa and 15.4 KPa respectively, representing 16.2 % and 10.0 % error in comparison with the contact stresses obtained from the numerical models. Compression-flexure tests complemented the dynamic models. The steel reinforcement plates were observed to protect the pommel from delamination which was the principal failure mode of the wooden pommel; however, the reinforced pommel failed in flexure. From the simulations and tests performed, it was evident that there is a stiffness mismatch between the saddletree and the horseback which is undesirable. In addition to this significant conclusion, it has been shown that the steel reinforcement plates have a significant effect on the stiffness of the saddletree and do not protect it from iv failing. Hence, their continued use in the design and manufacturing of the English jumping saddletree is not recommended. Invaluable knowledge gained from the research allowed the definition of the performance attributes in a materials selection process ensuring the choice of potential material candidates to guarantee the optimal performance of the saddletree. Finally, the findings were incorporated in the development of a new concept design for next generation English saddletrees. v ACKNOWLEDGEMENT I am sincerely grateful to Professor F. Richard Hall, whose invaluable contribution, encouragement, and support facilitated the development of my understanding of the subject. My appreciation also goes to Professor A. Pollard and Phil Wilson for their contribution; to the Centre of Engineering Excellence and the School of Technology (formerly known as SEBE) for providing some financial support for my research. I am grateful to Julian Spence for providing the initial training on the use of the simulation software and his support in ensuring access to relevant software, and to the staff of MSC.Software for their support. I would like to express my appreciation to Professor Kevin Kibble, Colin Durnall, and Kevin Worrall for their assistance in performing the laboratory experiments. In addition, I wish to recognise Bob Wood at Loughborough University for his contribution at the conception of the research; Ian Rae at Lariot, Frank Baines at Frank Baines Saddlery, and, Duncan Kent at E. Jeffries & Sons Ltd for their support. I am without a doubt grateful to my wife, children, brother, and sisters for their understanding and support. I recognise and appreciate their tolerance during this period. Finally, I express my gratitude to all those who supported and encouraged me to complete this research study. vi TABLE OF CONTENT ABSTRACT iii ACKNOWLEDGEMENTS vi TABLE OF CONTENTS vii LIST OF FIGURES xi LIST OF TABLES xvi NOMENCLATURE xvii CHAPTER 1 INTRODUCTION 1 1.1 Background information 1 1.2 The saddletree 2 1.3 Evolution of the saddletree design 3 1.4 Research drivers 6 1.4.1 Economic factors 6 1.4.2 Technological factors 7 1.5 Aim and objectives 8 1.6 Research framework 12 1.7 Structure of the thesis 13 CHAPTER 2 LITERATURE REVIEW 16 2.1 Design 16 2.2 Horseback movements 19 2.3 Saddlery and horseback interaction 23 2.4 Saddle fitting 24 2.5 Pressure mapping 24 2.5.1 Validity, accuracy, and repeatability 25 2.5.2 Display properties 27 2.5.3 Pressure mapping and finite element analysis 28 2.6 Material properties of wood 28 2.6.1 Elastic constants 28 2.6.2 Failure prediction 31 2.7 Finite element modelling 33 2.7.1 Introduction to FEM 33 2.7.2 Mesh generation 34 2.7.3 Element type 35 2.7.4 Ply lay-up 35 2.7.5 Convergence 36 2.7.6 Finite element analysis – parametric studies 37 2.7.7 Explicit and implicit dynamic transient analysis 38 2.7.8 Impact analysis – parametric studies 40 2.7.9 Results validation 42 2.8 Reverse engineering 43 CHAPTER 3 PREPARATIONS FOR FINITE ELEMENT ANALYSIS (FEA) 44 3.1 Introduction 44 3.2 Geometric modelling 44 vii 3.2.1 Reverse engineering 45 3.2.2 Data migration from CAD to FEA 49 3.3 Laminate modelling 52 3.3.1 Finite elements for modelling laminates 55 3.3.2 Example – Layerwise or conventional 3-D elements 57 3.3.2.1 Geometry 57 3.3.2.2 Load and boundary conditions 58 3.3.2.3 Material properties 58 3.3.2.4 Results 59 3.3.2.5 Hand calculations 63 3.3.2.6 Discussion 65 3.4 Summary 66 CHAPTER 4 SIMULATING BENDING IN THE SADDLETREE 67 4.1 Introduction 67 4.2 Parameters investigated 67 4.3 Pre-processing 68 4.3.1 Geometric models 69 4.3.2 Material properties 70 4.3.3 Load and boundary conditions 70 4.3.4 Element and element properties 73 4.4 MSC.Marc solution parameters 74 4.5 Post-processing 75 4.5.1 Point load 76 4.5.1.1 Saddletree (wood only) 76 4.5.1.2 Reinforced saddletree 77 4.5.1.3 Reinforced saddletree (excluding spring steel) 80 4.5.2 Uniformly Distributed Loading (UDL) 82 4.5.2.1 Saddletree (wood only) 82 4.5.2.2 Reinforced saddletree 83 4.5.2.3 Reinforced saddletree (excluding spring steel) 86 4.6 Discussion of results 88 4.6.1 The effect of the steel reinforcement on the wood only product 89 4.6.2 The effect of excluding the pair of spring steel 90 4.6.3 The effect of varying the saddletree wood thickness 92 4.6.4 The effect of loading the cantle region of the saddletree on the pommel 94 4.7 Summary 95 CHAPTER 5 SIMULATING BENDING IN THE SADDLETREE II 97 5.1 Introduction 97 5.2 Parameters investigated 100 5.3 Determining the method of loading 100 viii 5.3.1 Validation of ConforMat measurements 101 5.3.2 Contour mapping 102 5.4 Pre-processing 105 5.5 Post-processing 106 5.5.1 Model deflection 106 5.5.2 20 % 90 degrees and 80 % 0 degrees stacking sequence 110 5.5.3 40 % 90 degrees and 60 % 0 degrees stacking sequence 112 5.5.4 60 % 90 degrees and 40 % 0 degrees stacking sequence 117 5.5.5 80 % 90 degrees and 20 % 0 degrees stacking sequence 122 5.6 Discussion of results 124 5.6.1 The effects of reinforcing the saddletree on the stiffness of the saddletree 125 5..6.2 The effects of stacking sequence on the stiffness of the saddletree 126 5.7 Summary 129 CHAPTER 6 IMPACT SIMULATION OF THE SADDLETREE POMMEL 130 6.1 Introduction 130 6.2 Parameters investigated 131 6.3 Pre-processing 131 6.3.1 Geometric models 131 6.3.2 Material properties 132 6.3.3
Details
-
File Typepdf
-
Upload Time-
-
Content LanguagesEnglish
-
Upload UserAnonymous/Not logged-in
-
File Pages244 Page
-
File Size-