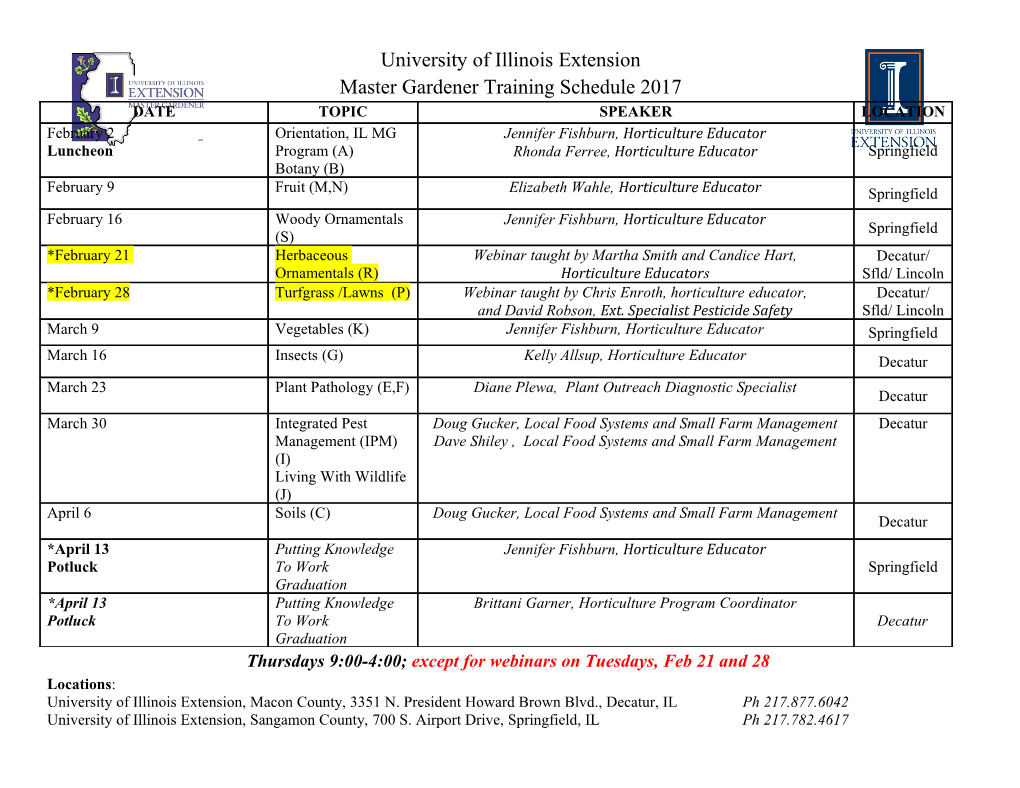
Article Anaerobic endosymbiont generates energy for ciliate host by denitrification https://doi.org/10.1038/s41586-021-03297-6 Jon S. Graf1 ✉, Sina Schorn1, Katharina Kitzinger1,2, Soeren Ahmerkamp1, Christian Woehle3, Bruno Huettel3, Carsten J. Schubert4, Marcel M. M. Kuypers1 & Jana Milucka1 ✉ Received: 10 December 2019 Accepted: 27 January 2021 Mitochondria are specialized eukaryotic organelles that have a dedicated function in Published online: 3 March 2021 oxygen respiration and energy production. They evolved about 2 billion years ago from Open access a free-living bacterial ancestor (probably an alphaproteobacterium), in a process Check for updates known as endosymbiosis1,2. Many unicellular eukaryotes have since adapted to life in anoxic habitats and their mitochondria have undergone further reductive evolution3. As a result, obligate anaerobic eukaryotes with mitochondrial remnants derive their energy mostly from fermentation4. Here we describe ‘Candidatus Azoamicus ciliaticola’, which is an obligate endosymbiont of an anaerobic ciliate and has a dedicated role in respiration and providing energy for its eukaryotic host. ‘Candidatus A. ciliaticola’ contains a highly reduced 0.29-Mb genome that encodes core genes for central information processing, the electron transport chain, a truncated tricarboxylic acid cycle, ATP generation and iron–sulfur cluster biosynthesis. The genome encodes a respiratory denitrifcation pathway instead of aerobic terminal oxidases, which enables its host to breathe nitrate instead of oxygen. ‘Candidatus A. ciliaticola’ and its ciliate host represent an example of a symbiosis that is based on the transfer of energy in the form of ATP, rather than nutrition. This discovery raises the possibility that eukaryotes with mitochondrial remnants may secondarily acquire energy-providing endosymbionts to complement or replace functions of their mitochondria. Eukaryotic life evolved at least around 1–1.9 billion years ago5,6, after have independently evolved from mitochondria in evolutionarily dis- the gradual oxygenation of the atmosphere of the Earth (which started tant organisms18,19. Some ciliates that contain hydrogenosomes also about 2.4 billion years ago7,8). The ensuing diversification of eukary- contain endosymbiotic methanogenic archaea that scavenge the pro- 20 otes has been attributed to their ability to harvest copious amounts duced H2, which thereby increases the energy yield of the reaction . of energy from the respiration of oxygen, an abundant, high-potential In contrast to strict anaerobes that exclusively ferment, some fac- electron acceptor. Oxygen respiration is performed in mitochondria, ultatively anaerobic eukaryotes can perform anaerobic respiration21. which are specialized subcellular organelles that arose from a free-living Nitrate and/or nitrite reduction has previously been demonstrated for prokaryotic organism through endosymbiosis9. During the evolution some foraminifera and Gromiida22–24, fungi25,26 and the ciliate Loxodes27. towards the contemporary organelle, the endosymbiont has either lost The underlying molecular mechanism of eukaryotic denitrification and its genes or transferred them to the host genome; mitochondria have energy conservation is largely unknown. Here we report the discovery kept only a small subset of genes10 that pertain to translation and the of an obligate endosymbiont of an anaerobic ciliate that provides its electron transport chain11,12. host with energy derived from denitrification. Some eukaryotes that inhabit anoxic environments do not contain aerobic mitochondria. Originally thought to be ‘ancestral’13, all of these amitochondriate eukaryotes in fact evolved secondarily from Anaerobic ciliates with bacterial endosymbionts predecessors that had aerobic mitochondria14; they possess highly We investigated Lake Zug, an approximately 200-m deep and perma- reduced mitochondria-related organelles or at least contain genes of nently stratified freshwater lake that is located in Switzerland. Lake Zug mitochondrial origin in their nuclear genomes15. Throughout the course contains deep waters that remain permanently anoxic and replete in of evolution, anaerobic unicellular protists have lost the capacity to nitrate, which is a feature that is characteristic of many stratified fresh and generate ATP via oxidative phosphorylation (with some exceptions16,17). marine waters depleted in oxygen. The thickness of the anoxic hypolim- Instead, these eukaryotes generate ATP from fermentation through nion varied between 40 and 100 m over a period of 5 years (2016—2020) 18 substrate-level phosphorylation, coupled to the formation of H2 (ref. ). (Extended Data Fig. 1). In 2018, oxygen was undetectable below a depth of This reaction takes place in the cytoplasm or—more commonly—in 160 m, and a decrease in NOx (mainly nitrate) concentration below the base hydrogenosomes. Hydrogenosomes are specialized organelles, which of the oxycline indicated ongoing denitrification (Extended Data Fig. 1). 1Max Planck Institute for Marine Microbiology, Bremen, Germany. 2Division of Microbial Ecology, Centre for Microbiology and Environmental Systems Science, University of Vienna, Vienna, Austria. 3Max Planck Genome Centre Cologne, Max Planck Institute for Plant Breeding Research, Cologne, Germany. 4Surface Waters - Research and Management, Eawag, Kastanienbaum, Switzerland. ✉e-mail: [email protected]; [email protected] Nature | Vol 591 | 18 March 2021 | 445 Article acbd ‘Ca. A. ciliaticola’ Cilia DAPI Cilia MAC e f Plagiopylida Legionellales (83.0–84.6%, n = 12) Black Sea redoxcline clone (HM749917) Francisellales (79.6–82.0%, n = 8) Guaymas Basin HV sediment clone (JX268867) Guaymas Basin HV sediment clone (JX268824) Activated sludge metag. contig (FPLP01004190) Guaymas Basin HV sediment clone (JX268902) Activated sludge metag. contig (FPLP01007300) Guaymas Basin HV sediment clone (JX268831) Waste water clone (JF828756) B Guaymas Basin HV sediment clone (JX268891) Hypersaline mat clone (JN428441) (89.5–92.9%) Guaymas Basin HV sediment clone (JX268813) Marine denitrication system clone (AY835674) Baltic Sea redox cline clone (KC492846) Hydrate Ridge cold seep sediment clone (KT346312) Gulf of Mexico sediment clone (KX172462) Guaymas Basin HV sediment clone (JX268912) Feitsui reservoir clone (AB930564) Guaymas Basin HV sediment clone (JX268865) Feitsui reservoir clone (AB930693) Azoamicus’ Guaymas Basin HV sediment clone (JX268913) ‘Ca. Azoamicus ciliaticola’ A Lake Zug single ciliate S3 Ca. Lake Pavin clone eub62A3 (GQ390243) (98.5–99.1%) ‘ Lake Zug, metagenome-assembled Plagiopylea Lake Kivu metag. contig (Ga0116204_10069046) Lake Zug multiple ciliates C10 Northeast subarctic Pacic Ocean clone (HQ674626) Lake Zug multiple ciliates C5 Lake Zug single ciliate S1 Delaware Bay clone (EU800820) NKB5 Lake Zug single ciliate S4 Nankai Trough sediment clone NKB5 (AB013257) (86.2–86.3%) Benthic diatom lm clone (KT201586) Black Sea redoxcline clone (HM749918) ‘Ca. Berkiella’ (84.1–86.5%, n = 5) Marine anoxic sinkhole mat clone (JF971654) Lake Zug single ciliate S2 Coxiellales (82.0–84.4%, n = 10) Guaymas Basin HV sediment clone (JX268874) Guaymas HV sediment clone (JX268870) Diplorickettsiales (79.5–82.5%, n = 6) 0.05 0.05 Epalxella antiquorum (EF014286) Framvaren Fjord clone (EF526988) Epalxellidae Wastewater treatment plant clone (AB902303) Fig. 1 | Visualization and phylogenetic affiliation of ‘Ca. A. ciliaticola’ and the ‘Ca. Azoamicus’ group are indicated. The sequence similarities of respective its ciliate host. a, Representative scanning electron microscopy image of a groups to the 16S rRNA gene sequence of ‘Ca. A. ciliaticola’ are shown in ciliate from the anoxic hypolimnion of Lake Zug (February 2020, depth of parentheses. The full tree is shown in Extended Data Fig. 4. Taxonomic groups 186 m). Scale bar, 5 μm. b–d, Differential interference contrast image (b) and were assigned on the basis of SILVA taxonomy45. Metag., metagenome. f, Ciliate confocal laser scanning microscopy image (c) of a ciliate after hybridization with 18S rRNA gene sequence-based maximum likelihood phylogenetic tree of the a ‘Ca. Azoamicus’-specific oligonucleotide probe (eub62A3_813) (yellow) and class Plagiopylea based on EukRef-Ciliophora reference alignment30. Sequences counterstaining with DAPI (blue). d, Overlay of fluorescence and differential of ciliates from Lake Zug (in bold) were amplified from individual ciliates (S1–S4) interference contrast images. The macronucleus (MAC) with attached or combined ciliates (C5 and C10). Stars denote positive identification of a ‘Ca. A. micronucleus (arrowhead) and putative food vacuoles of the ciliate are outlined. ciliaticola’ 16S rRNA sequence amplified from the same ciliate: black, ‘Ca. A. Scale bars, 5 μm. e, 16S rRNA gene sequence-based maximum likelihood ciliaticola’ 16S rRNA gene sequence verified by Sanger sequencing; grey, positive phylogenetic tree of ‘Ca. A. ciliaticola’ (bold denotes sequence derived from band on gel. For both trees, bootstrap values are shown as circles at the circular metagenome-assembled genome) and members of related respective nodes, and indicate bootstrap support of >70% (grey) or >90% (black) gammaproteobacterial orders and environmental clades. Subgroups A and B of out of 100 resamplings. Scale bars, 0.05 nucleotide substitutions per site. We observed a high abundance of motile unicellular eukaryotes a general bacteria-specific oligonucleotide probe (EUB-I) (Supplemen- throughout the anoxic layer (Fig. 1, Extended
Details
-
File Typepdf
-
Upload Time-
-
Content LanguagesEnglish
-
Upload UserAnonymous/Not logged-in
-
File Pages23 Page
-
File Size-