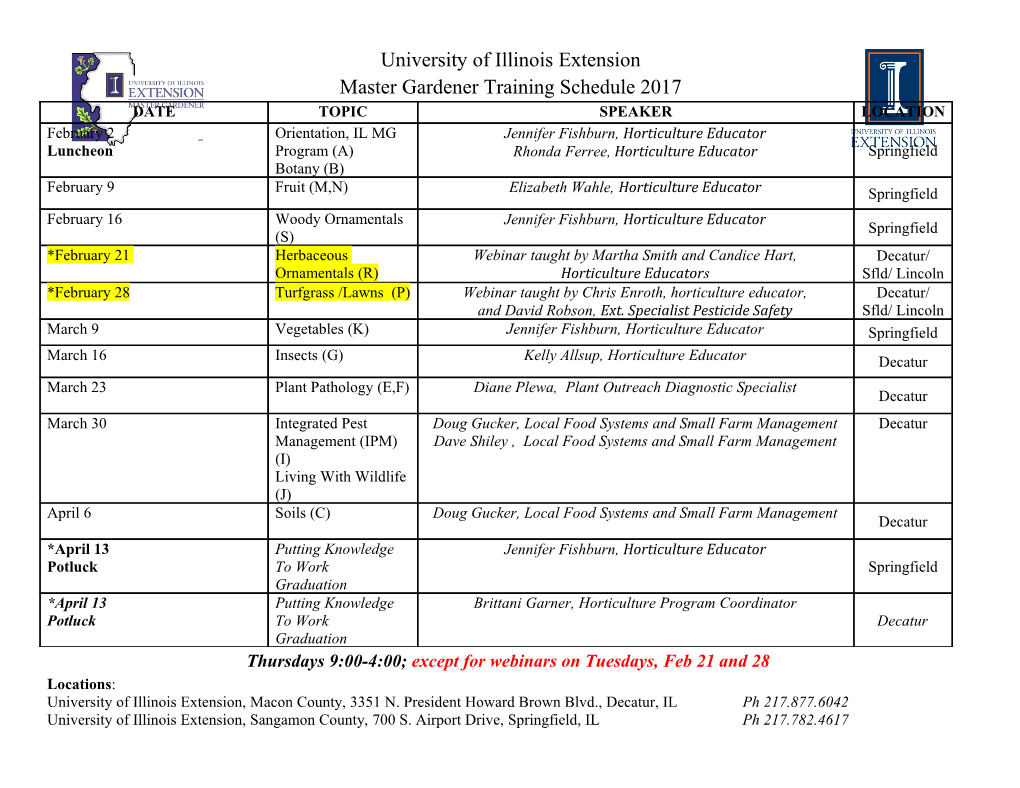
GENE DISPERSAL IN TROPICAL TREES: ECOLOGICAL PROCESSES AND GENETIC CONSEQUENCES by Na Wei A dissertation submitted in partial fulfillment of the requirements for the degree of Doctor of Philosophy (Ecology and Evolutionary Biology) in the University of Michigan 2015 Doctoral Committee: Associate Professor Christopher W. Dick, Chair Professor Deborah E. Goldberg Associate Professor Inés Ibáñez Assistant Professor Timothy Y. James © Na Wei 2015 ACKNOWLEDGMENTS Many people need to be acknowledged here. First, I would like to thank my advisor, Chris Dick, for his support all the way through my doctoral dissertation work. I would also like to thank my dissertation committee of Deborah Goldberg, Inés Ibáñez and Tim James for their intellectual input into my dissertation research. In addition, I must emphasize that most of work in this dissertation would not have been possible without my collaborators Matteo Detto, Marjolein Bruijning, Jordan Bemmels, Andrew Lowe and Michael Gardner. I am very grateful to the Panamanian field technicians, Yuriza Guerrero, Anayansi Cerezo and Oldemar Valdez, for assisting me in surveying, mapping and collecting plant samples in the tropical rainforests on Barro Colorado Island, Panama. I thank my husband, Yubo Xia, for helping me with the molecular laboratory work. Lastly, I would like to acknowledge with gratitude, the funding support provided by a CTFS-ForestGEO Grant from the Center for Tropical Forest Science and Smithsonian Tropical Research Institute, an International Research Award and a Graduate Student Research Grant from Rackham Graduate School, a Winifred B. Chase Fellowship from Matthaei Botanical Gardens and Nichols Arboretum, an Angeline B. Whittier Fellowship and an Emma J. Cole Fellowship from the Department of Ecology and Evolutionary Biology, as well as the financial support provided by a Barbour Scholarship from Rackham Graduate School and fellowships from EEB department. ii TABLE OF CONTENTS ACKNOWLEDGMENTS ii LIST OF FIGURES v LIST OF TABLES vii ABSTRACT ix CHAPTER I. Introduction 1 II. The effects of read length, quality and quantity on microsatellite discovery and primer development: from Illumina to PacBio 15 Abstract 15 Introduction 17 Materials and Methods 21 Results 27 Discussion 33 Appendix A Polymorphic microsatellite loci for Virola sebifera (Myristicaceae) derived from shotgun 454 pyrosequencing 60 iii Appendix B Characterization of twenty-six microsatellite markers for the tropical pioneer tree species Cecropia insignis Liebm. (Urticaceae) 69 Appendix C Polymorphic microsatellite markers for a wind-dispersed tropical tree species, Triplaris cumingiana (Polygonaceae) 76 III. Seed dispersal drives spatial genetic patterns in tropical trees 87 Abstract 87 Introduction 89 Methods 92 Results 101 Discussion 104 IV. Frequent long-distance seed and pollen dispersal and their genetic impacts in tropical trees 140 Abstract 140 Introduction 142 Materials and Methods 146 Results 153 Discussion 160 V. Conclusions 198 iv LIST OF FIGURES FIGURE 2.1 Frequency distribution of CCS read lengths generated by 500-bp genomic shotgun circular consensus sequencing 43 2.2 Base quality scores of CCS reads before (untrimmed) and after (trimmed) quality control 44 2.3 Motif length-specific microsatellite loci identified from quality-controlled CCS reads 45 2.4 The effects of read length on microsatellite yield (a–b), genomic redundancy detection (c), and primer design success rate (d) 46 2.5 Simulations of microsatellite amplification rate in relation to sequencing errors 47 2.6 The effect of quality control on microsatellite locus amplification 48 2S.1 Frequency distribution of mean quality score of individual CCS reads before (untrimmed) and after quality control (trimmed) 51 2S.2 Homopolymer lengths of CCS reads 52 2S.3 The number of CCS reads generated by a single SMRT cell 53 2S.4 Negative correlation between sequence quality and sequence length in raw CCS reads 54 2S.5 Positive correlation between sequence quality and sequence length in post-QC CCS reads 55 2S.6 Positive correlation between homopolymer length and raw CCS read length 56 2S.7 Base position GC content of CCS reads before (black) and after quality control (grey) 57 3.1 Spatial genetic structure in seedling banks (a) and adults trees (b) of the four 114 v tropical tree species 3.2 Spatial genetic structure of seedlings to female trees and to male trees 115 3.3 Posterior distribution of median seed dispersal distance inferred from the spatial genetic structure of seedlings using the approximate Bayesian computation method 116 3.4 Posterior distribution of median pollen dispersal distance inferred from the spatial genetic structure of seedlings using the approximate Bayesian computation method 117 3.5 Spatial genetic structure of seedlings as a function of median seed and pollen dispersal distance 118 3S.1 Correlogram of spatial genetic structure 132 3S.2 Spatial patterns of seedlings and adult trees 133 3S.3 Spatial genetic relatedness of seedlings to the subset of female and male trees 134 3S.4 Posterior distribution of mating neighborhood size 135 3S.5 Validating ABC inference of median seed dispersal distance 136 3S.6 Validating ABC inference of Nm 137 3S.7 SGS as a function of median seed and pollen dispersal distance 138 4.1 Frequency distribution of seed dispersal distances based on parentage inference 178 4.2 Frequency distribution of pollen dispersal distances based on parentage inference 179 4.3 Comparisons between seed and pollen dispersal distances 180 4S.1 Frequency distribution of pollen-mediated gene dispersal distances 194 4S.2 Comparisons between distances of father trees to mother trees and distances to the tenth nearest female neighbors 195 4S.3 Comparisons between seed and pollen-mediated gene dispersal 196 vi LIST OF TABLES TABLE 2.1 Sequencing capacity and microsatellite throughput of 500-bp genomic shotgun circular consensus sequencing using four SMRT cells per species 49 2.2 Cross-platform comparisons of next-generation sequencing (NGS) use in microsatellite development 50 2S.1 Simulations of microsatellite detection effectiveness in relation to read length when sequencing errors were not introduced 58 2S.2 Simulations of microsatellite detection effectiveness in relation to read length when PacBio CCS error profiles were used 59 A1 Characteristics of ten polymorphic SSR markers developed in Virola sebifera 67 A2 Summary statistics of SSR marker polymorphism screened in 42 V. sebifera individuals located in the 50-ha Forest Dynamics Plot on Barro Colorado Island, Panama 68 B1 Characteristics of 26 microsatellite markers developed in Cecropia insignis 74 C1 Characteristics of twelve polymorphic microsatellite markers developed in Triplaris cumingiana 83 C2 Summary statistics of microsatellite marker polymorphism tested on 47 reproductively mature trees of Triplaris cumingiana 84 C3 Additional 14 polymorphic microsatellite markers of Triplaris cumingiana screened on 12 individuals sampled from the 50-ha Forest Dynamics Plot on Barro Colorado Island, Panama 85 3S.1 Allelic richness of microsatellite markers 130 3S.2 Relative bias and relative root mean square error of ABC parameter estimates 131 vii 4.1 Median seed and pollen dispersal distance 176 4.2 Simulated genetic impacts of near vs. far-tail seed and pollen dispersal 177 4S.1 Genotyping error rates assumed in parentage inference using COLONY program 188 4S.2 Estimated parameters of seed dispersal models–GSMi, GSM, SSMi and SSM 189 4S.3 Estimated parameters of pollen dispersal model CSMi 190 4S.4 Simulated genetic impacts of near vs. far-tail seed and pollen dispersal following algorithm 1 in Appendix 4S.2 191 4S.5 Simulated genetic impacts of near vs. far-tail seed and pollen dispersal following algorithm 2 in Appendix 4S.2 192 4S.6 Simulated genetic impacts of near vs. far-tail seed and pollen dispersal following algorithm 3 in Appendix 4S.2 193 viii ABSTRACT Tropical trees constitute an ecologically important functional group in terrestrial ecosystems because of the essential roles that they play in sustaining biodiversity and carbon storage. The persistence and evolutionary potentials of tropical trees are, however, increasingly threatened by human-induced rapid changes in abiotic and biotic environments. For long-lived forest trees, gene dispersal by seeds and pollen is critical for tracking shifting climatic niches and for maintaining genetic variation needed to adapt to changing environments. Understanding the potential responses of tropical trees to environmental changes depends in part upon quantifying the rates of seed and pollen dispersal. This dissertation aims to quantify the spatial extent and magnitude of seed and pollen dispersal and their respective genetic impacts in a comparative context, by focusing on four Neotropical tree species that have distinct dispersal and pollination syndromes and life-history strategies. By using parentage inference and inverse modeling, I found that long-distance gene dispersal by seeds is common in these vertebrate-dispersed tropical trees, in which models predicted 1–18% of dispersal events exceeding 1 km. This fraction of pollen dispersal >1 km could reach 10–20% in these species. Furthermore, simulations with gene dispersal distances realistically represented suggest that seed and pollen dispersal limitation can lead to genetic diversity loss in tropical tree populations. By examining the respective genetic impacts of seed vs. pollen dispersal, I found that seed dispersal is the primary force driving
Details
-
File Typepdf
-
Upload Time-
-
Content LanguagesEnglish
-
Upload UserAnonymous/Not logged-in
-
File Pages218 Page
-
File Size-