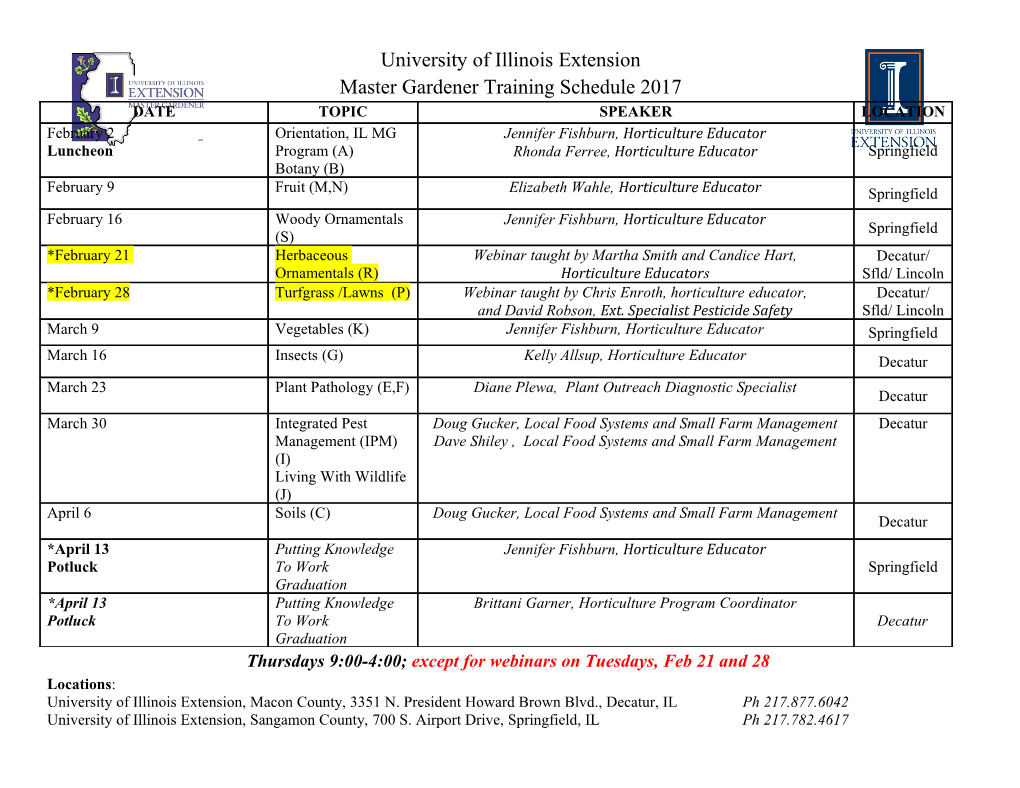
THE JOURNAL OF BIOLOGICAL CHEMISTRY Vol. 278, No. 21, Issue of May 23, pp. 19463–19472, 2003 © 2003 by The American Society for Biochemistry and Molecular Biology, Inc. Printed in U.S.A. Structures of Shikimate Dehydrogenase AroE and Its Paralog YdiB A COMMON STRUCTURAL FRAMEWORK FOR DIFFERENT ACTIVITIES* Received for publication, January 24, 2003, and in revised form, March 10, 2003 Published, JBC Papers in Press, March 12, 2003, DOI 10.1074/jbc.M300794200 Gurvan Michel‡, Aleksander W. Roszak§¶,Ve´ronique Sauve´‡, John Maclean§, Allan Matte‡, John R. Coggins¶, Miroslaw Cygler‡ʈ, and Adrian J. Lapthorn§** From the ‡Biotechnology Research Institute, NRC Macromolecular Structure Group, Montreal, Quebec H4P 2R2, Canada and the §Department of Chemistry and ¶Division of Biochemistry and Molecular Biology, Institute of Biomedical and Life Sciences, University of Glasgow, Glasgow G12 8QQ, Scotland, United Kingdom Shikimate dehydrogenase catalyzes the fourth step of opment of nontoxic herbicides (3), as well as antimicrobial (4) the shikimate pathway, the essential route for the bio- and antiparasite (2) agents. The sixth step in the pathway, synthesis of aromatic compounds in plants and micro- catalyzed by 5-enolpyruvylshikimate-3-phosphate synthase, organisms. Absent in metazoans, this pathway is an at- has already been successfully targeted, with the development tractive target for nontoxic herbicides and drugs. of glyphosate, a broad spectrum herbicide (5). However, after Escherichia coli expresses two shikimate dehydrogen- 20 years of extensive use, glyphosate-resistant weeds have ase paralogs, the NADP-specific AroE and a putative recently emerged (6), emphasizing the importance of maintain- enzyme YdiB. Here we characterize YdiB as a dual spec- ing target diversity. In order to design new inhibitors, crystal ificity quinate/shikimate dehydrogenase that utilizes ei- structures of several enzymes of the shikimate pathway have ther NAD or NADP as a cofactor. Structures of AroE and been elucidated recently: 3-dehydroquinate synthase (7), type I YdiB with bound cofactors were determined at 1.5 and and II dehydroquinases (8), type I and II shikimate kinases (9, 2.5 Å resolution, respectively. Both enzymes display a 10), and 5-enolpyruvylshikimate-3-phosphate synthase (11), similar architecture with two ␣/ domains separated by catalyzing the second, third, fifth, and sixth steps of the path- a wide cleft. Comparison of their dinucleotide-binding domains reveals the molecular basis for cofactor speci- way, respectively. ficity. Independent molecules display conformational Shikimate dehydrogenase (EC 1.1.1.25) catalyzes the fourth flexibility suggesting that a switch between open and reaction in the shikimate pathway, the NADP-dependent re- closed conformations occurs upon substrate binding. duction of 3-dehydroshikimate to shikimate (Fig. 1A). Whereas Sequence analysis and structural comparison led us to dehydrogenases usually form oligomers, shikimate dehydro- propose the catalytic machinery and a model for 3-de- genase, coded by the gene aroE in Escherichia coli, is present as hydroshikimate recognition. Furthermore, we discuss a monomer in most bacteria (12, 13). In higher organisms this the evolutionary and metabolic implications of the pres- activity is part of a multifunctional enzyme. In plants shiki- ence of two shikimate dehydrogenases in E. coli and mate dehydrogenase is associated with type I dehydroquinase other organisms. to form a bifunctional enzyme (14), whereas in fungi, such as Neurospora crassa, this enzyme forms the fifth domain of the pentafunctional AROM polypeptide, which catalyzes five of The shikimate pathway, which links metabolism of carbohy- seven steps of the shikimate pathway (15). However, the mo- drates to biosynthesis of aromatic compounds, is essential to lecular basis of 3-dehydroshikimate recognition and enzymatic plants, bacteria, and fungi (1) as well as apicomplexan para- reduction is not known. sites (2). This seven-step metabolic route leads from phos- Although in E. coli AroE is strictly specific for shikimate, phoenolpyruvate and erythrose 4-phosphate to chorismate, the some fungal shikimate dehydrogenases can also utilize quinic common precursor for the synthesis of folic acid, ubiquinone, acid as a substrate. This compound, which differs from shi- vitamins E and K, and aromatic amino acids (1). This pathway kimic acid only by the addition of a hydroxyl group at C-1 (Fig. is absent in metazoans, which must obtain the essential amino 1B), is the precursor to the ubiquitous plant secondary product acids phenylalanine and tryptophan from their diet. Therefore, chlorogenate (1). To date, two independent families of quinate/ enzymes of this pathway are important targets for the devel- shikimate dehydrogenases have been identified. The first con- sists of NAD-dependent dehydrogenases (16), and the second * This work was supported in part by Canadian Institutes of Heath consists of membrane-associated dehydrogenases that utilize Research Grant 200103GSP-90094-GMX-CFAA-19924 (to M. C.). The pyrrolo-quinoline-quinone as a cofactor (17). Both types of de- costs of publication of this article were defrayed in part by the payment hydrogenases are involved in the catabolic quinate pathway, of page charges. This article must therefore be hereby marked “adver- tisement” in accordance with 18 U.S.C. Section 1734 solely to indicate which allows growth of microorganisms with quinate as the this fact. sole carbon source by its conversion into protocatechuate and The atomic coordinates and structure factors (code 1NYT and 1O9B) subsequent metabolism by the -ketoadipate pathway (16, 17). have been deposited in the Protein Data Bank, Research Collaboratory By using BLAST (18), ϳ130 sequences, mostly annotated as for Structural Bioinformatics, Rutgers University, New Brunswick, NJ putative shikimate dehydrogenases, can be identified as homol- (http://www.rcsb.org/). ʈ To whom correspondence may be addressed: Biotechnology ogous to AroE through the entire length of the gene, thereby Research Institute, NRC Macromolecular Structure Group, 6100 defining the shikimate dehydrogenase (SDH)1 family. It also Royalmount Ave., Montreal, Quebec H4P 2R2, Canada. E-mail: includes the NAD-dependent quinate/shikimate dehydroge- [email protected]. ** To whom correspondence may be addressed: Dept. of Chemistry, University of Glasgow, Glasgow G12 8QQ, Scotland, UK. E-mail: 1 The abbreviations used are: SDH, shikimate dehydrogenase; DTT, [email protected]. dithiothreitol; r.m.s.d., root mean square deviation. This paper is available on line at http://www.jbc.org 19463 19464 Crystal Structure of Shikimate Dehydrogenases NaCl, 5% (w/v) glycerol, 5 mM DTT. Initial crystals were obtained by sparse-matrix screening (Hampton Research, Laguna Niguel, CA) by hanging drop vapor diffusion. The best crystals grew at 21 °C from droplets containing 2 l of protein solution and 2 l of reservoir solution (0.8 M KH2PO4, 0.8 M NaH2PO4, 0.1 M Hepes, pH 7.5, 2 mM NADH) and reached their maximal size after 2 days. The crystals are hexagonal, ϭ ϭ ϭ ␣ ϭ space group P64 with cell dimensions a b 157.87 Å, c 40.01 Å,  ϭ 90°, ␥ ϭ 120° with two molecules in the asymmetric unit. Structure Solution and Refinement—Native data were collected from cryo-cooled AroE crystals to 1.5 Å resolution at station 9.6 at the Daresbury SRS using a (ADSC) Quantum-4 charge-coupled device de- tector. Data were collected in-house, by using a MacScience DIP2000 detector on various crystals soaked with heavy atom solutions. Crystals soaked with Hg(CN)2 produced the only usable derivative that was isomorphous to the native crystals. This derivative was collected at a wavelength to maximize the anomalous signal on station 9.5 at the Daresbury SRS using a Mar charge-coupled device detector in a SIRAS experiment. All data were indexed and processed with the HKL suite (21), the cell dimensions and space group shown in Table I. Further FIG.1.The reactions catalyzed by shikimate (A) and quinate processing was carried out using programs from the CCP4 package (22). (B) dehydrogenases. From the anomalous Patterson map it was possible to identify 13 mercury sites using SHELX-90 (23), which were refined in Mlphare against the native 1.5-Å data to maximize the isomorphous signal. nases, whereas the pyrrolo-quinoline-quinone-dependent en- Phase refinement and extension was performed using the program DM zymes compose a different protein family. This family displays with solvent flattening and histogram matching. Averaging was at- no significant sequence similarity with any other NAD(P)-de- tempted but was unsuccessful because of the large variation in confor- pendent dehydrogenases, therefore constituting a distinct de- mation in the independent molecules in the asymmetric unit. Refine- hydrogenase family. Analysis of the complete genome of E. coli ment was carried out using the maximum likelihood refinement K12 and pathogenic O157:H7 strains has revealed the presence program REFMAC (24). Five percent of the data were randomly set aside as test data for calculation of Rfree. The structure was built of a gene of unknown function, ydiB, which shares 25% se- automatically using the program ARP/WARP (25) and assembled into quence identity with aroE. Thus, AroE and YdiB are paralogs, the four independent chains that were Ͼ90% complete. Manual correc- the only two proteins from the SDH family present in E. coli. tion of the structure and model building and addition of solvent was Here we report the biochemical characterization of YdiB and performed using modules within the program QUANTA (Accelrys Inc.). Nine iterations of refinement and manual rebuilding with the addition demonstrate that it is a quinate/shikimate dehydrogenase that ϩ can utilize either NAD or NADP as a cofactor. We have deter- of molecules of NADP , sulfate, glycerol, and DTT with the application of individual anisotropic temperature factors in the final stages of mined crystal structures of both these enzymes, AroE at 1.5 Å refinement resulted in a model with the final Rwork of 14.7% and Rfree of and YdiB at 2.5 Å resolution.
Details
-
File Typepdf
-
Upload Time-
-
Content LanguagesEnglish
-
Upload UserAnonymous/Not logged-in
-
File Pages10 Page
-
File Size-