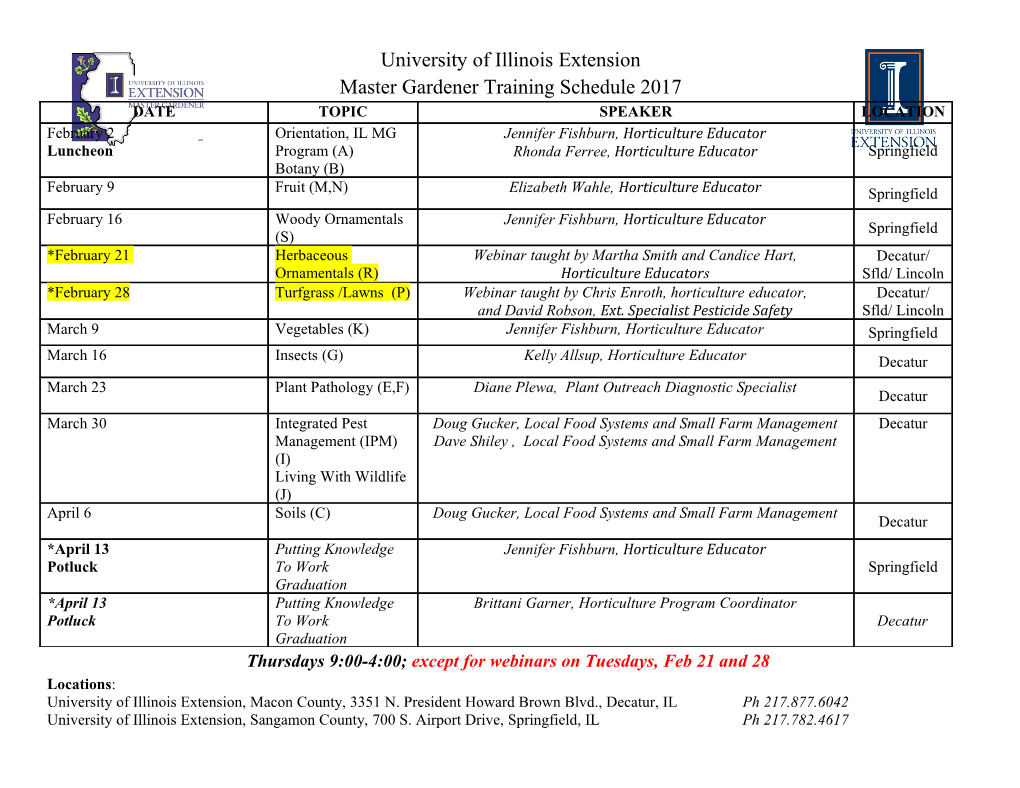
SYNTHESIS OF OLIGOESTERS FROM BIOBASED BUILDING BLOCKS BY CANDIDA ANTARCTICA LIPASE B David I. Habeych N. Thesis committee Thesis supervisor Prof. dr. G. Eggink Professor of Industrial Biotechnology Wageningen University Thesis co-supervisor Dr. ir. C. G. Boeriu Senior Scientist, Biobased Products Wageningen University Other members Prof. Dr. W.J.H. van Berkel Wageningen University Dr. Ir. H. Noorman DSM, Delft Dr. C.J.M. van den Heuvel Teijin Aramid, Arnhem Prof. Dr. Ir. M.W. Fraaije University of Groningen This research was conducted under the auspices of the VLAG Graduate School. BIOCATALYTIC SYNTHESIS OF CYCLIC ESTER OLIGOMERS FROM BIOBASED BUILDING BLOCKS David I. Habeych N. Thesis submitted in fulfillment of the requirements for the degree of doctor at Wageningen University by the authority of the Rector Magnificus Prof. dr. M.J. Kropff, in the presence of the Thesis Committee appointed by the Academic Board to be defended in public on Tuesday 13th of September 2011 at 1.30 p.m. in the Aula. David I. Habeych N. Biocatalytic synthesis of cyclic ester oligomers from biobased building blocks Thesis, Wageningen University, The Netherlands (2011) With summaries in English, Spanish, and Dutch ISBN: 978-90-8585-988-8 Dedicado a mi padre, mi madre y al gato CONTENTS Chapter 1. Introduction 9 Chapter 2. Biocatalytic synthesis of oligoesters from succinic acid 43 and 1,4‐butanediol using immobilized Candida antarctica lipase B Chapter 3. Biocatalytic synthesis of polyesters from sugar‐based 75 building blocks using immobilized Candida antarctica lipase B Chapter 4. Biocatalytic synthesis of oligoesters from alkyl and 107 furan derivatives using immobilized Candida antarctica Lipase B Chapter 5. Process strategies to improve the calb‐catalyzed 133 synthesis of ester oligomers from biobased building blocks Chapter 6. General discussion 169 Summary 197 Samenvatting 201 Resumen 207 Acknowledgements 213 Curriculum vitae 221 List of Publications 223 Training activities 225 INTRODUCTION 1. GENERAL INTRODUCTION 10 1. GENERAL INTRODUCTION 1. INTRODUCTION Polyesters are an important class of polymers with exceptional physical and mechanical properties and numerous applications. Polyester materials are used as fibres, plastics 11 and coating or as components of films, in composites and elastomers. Most of the polyesters applied today are built up from monomers derived from fossil fuel. Polyethylene terephtalate (PET), polybutylene succinate (PBS), polyacrylates are only few examples of largely used polyesters produced from petrochemical feedstocks. With oil resources forecasted to exhaust by year 2050, the permanent fluctuations of the oil price marked by political issues, increasing environmental awareness worldwide and the development of new technologies, there is growing interest for biobased polyesters produced from renewable feedstocks. Currently, there are only few biobased polyesters. Representative examples of natural occurring polyesters are polyhydroxyalkanoates (PHA) which are linear polyesters produced by microbial fermentation from sugars or lipids. Typical building blocks of PHA are 3 (or 4)‐hydroxy butyrate, 3‐hydroxy valerate, and 3‐hydroxy hexanoate. Polymers made of PHA were industrially marketed by ICI/Zeneca and later by Monsanto. The industrial production became unsustainable after 1995 (Gross and Kalra 2002). In the past decade much effort has been put to develop high production strains, to advance the technology for PHA production, to increase the production yield and to improve the downstream processing. Research on production of functionalized PHA with reactive groups in the aliphatic side chain is in progress. In 2005, Metabolix restarted the commercialization of microbial PHAs and currently develops transgenic crops to improve the production. Another example of a commercially produced biobased semisynthetic polyester is polylactic acid. The building blocks of PLA, L‐lactic acid, is nowadays directly obtained by fermentation of sugars using microorganism (i.e. lactobacillus sp.). Industrial 1. GENERAL INTRODUCTION manufacture of high molecular weight PLA is carried out via polycondensation (PC) (implemented by Mitsui Toatsu Chemicals) and ring‐opening polymerization (ROP) from the cyclic ester dimer (lactide) (implemented by Cargill). PLA is the first renewable 12 polymer produced on an industrial scale. In April 2002, Cargill opened PLA production plant with an estimated capacity of 150.000 tonne per year. PLA has properties similar with the conventional polymers polyethylene and polypropylene, and is completely biodegradable (compostable). PLA has a wide range of applications, such as fabrics production, disposable plastic materials. Blends of PLA have a good resistance to deformation and transparency, making them useful for disposable tableware, and packaging applications. More specialized products are sutures, stents, dialysis media, and drug delivery devices. The development of the technology to produce diversity of biobased building blocks is a way to increase the number of biobased polyesters with wide spectrum of chemical, physical and mechanical properties. This new generation of biobased polyesters will replace the petroleum derived polymers (Scheme 1). These new building blocks could eventually be associated with green, biocatalytic technologies for polyesterification. The latest is the topic of this thesis. 1. GENERAL INTRODUCTION 13 Scheme 1 Products obtained by renewable resources. Biorefinery products. (adapted from chemistry innovation website) 2. BIOBASED BUILDING BLOCKS USED IN THIS WORK. Biobased building blocks are obtained by biorefining of biomass (Scheme 1). From the multitude of compounds that are produced in the biorefinery, some 1. GENERAL INTRODUCTION of them are already produced industrially and have found industrial application for polyester synthesis, like L‐lactic acid and 1,3‐propanediol. For others, like succinic acid, the technology for their production is still under 14 development. Nevertheless, the availability of bio‐succinic acid will allow the production of a fully bio‐polybutylene succinate (PBS) (since the co‐monomer 1,4‐butanediol can also be produced from succinic acid) that can replace the petroleum‐based PBS that is currently commercialized. Also other compounds can be produced from C6 sugars that are emerging as building blocks for the polyester synthesis, namely, dianhydrohexitols and the furan‐based monomers, like furan‐2,5‐dicarboxylic acid (FDA), 5‐(hydroxymethyl)furan‐2‐ carboxylic acid (HMFA), and 2,5‐bis (hydroxyl methyl)furan (BHMF). These building blocks form the core of this thesis; therefore, we will focus below on some aspects of their production (Scheme 2). 2.1. 1,4‐Butanediol (BDO) and Succinic Acid (1,4‐Butanedioic Acid) Succinic acid and 1,4‐butanediol can be fully synthesized using sugar‐based feedstock by bacterial fermentation (Hofvendahl and Hahn–Hagerda 2000; Zeikus et al. 1999). A. succiniciproducens can utilize several substrates as carbon sources (Lee et al. 1999; Lee et al. 2001). High production yields (1.3 mole/mole) and low concentration of by‐products can be obtained in the synthesis of succinic acid by A. succiniciproducens using glycerol as substrate (Lee et al. 2001). 1. GENERAL INTRODUCTION 15 Scheme 2 Building blocks used in this work for the synthesis of biobased low molecular weight polyesters. A. succinogenes metabolizes carbon sources to succinic acid, acetic acid, and formic acid and tolerates a larger concentration of succinic acid than any other strain (Guettler et al. 1996a; Guettler et al. 1996b). One of the drawbacks of fermentation of A. succinogenes is the high concentrations of by‐products like acetic acid, propionic acid, and pyruvic acid which results in a high downstream processing costs. Succinic acid yield with variants of this strain is around 1 mol/mol glucose and has a productivity of 0.79 g.(L.h)‐1 (Guettler et al. 1996a). Recently, a metabolically engineered E. coli strain has been reported to produce aerobically 58.3 g.L–1 succinic acid with a yield of 0.85 mol/mol glucose. The synthesis of by‐products, however, could not be avoided (Lin et al. 2005a; Lin et al. 2005b). The purification of succinic acid from fermentative synthesis normally amounts more than 1. GENERAL INTRODUCTION 50% of the total production costs. Furthermore, the purification of succinic acid has been explored using electrodialysis, precipitation, and extraction with purities between 80 and 99% and efficiencies between 70 and 80% (Datta et al. 1992; Glassner and Datta 1992; 16 Huh et al. 2006). Succinic acid can be directly modified to other biobased building blocks or to precursors with a potential use at industrial scale. Three succinate derivatives with major applications are obtained through hydrogenation routes. These are ‐ butyrolactone (GBL), 1,4‐butanediol (BDO), and tetrahydrofuran (THF). From these three, BDO has a wide application as industrial solvent and recently it has been used as raw material for polyesters, such as polybutylene terephthalate and polybutylene succinate (GS Pla®, Bionolle®), resins, automotive, and electrical parts. The potential market for products based on succinic acid is estimated at 270 ktonne.yr‐1 (Willke and Vorlop 2004), while BDO has a global market of 1,300 ktonne.yr‐1 (Delhomme et al. 2009). 2.2. 1,4:3,6-Dianhydrohexitols (DAH) 1,4:3,6‐Dianhydrohexitol (DAH) is the name for 1,4:3,6‐dianhydro‐D‐glucitol or isosorbide; 1,4:3,6‐dianhydro‐D‐mannitol or isomannide; and 1,4:3,6‐dianhydro‐L‐iditol or
Details
-
File Typepdf
-
Upload Time-
-
Content LanguagesEnglish
-
Upload UserAnonymous/Not logged-in
-
File Pages226 Page
-
File Size-