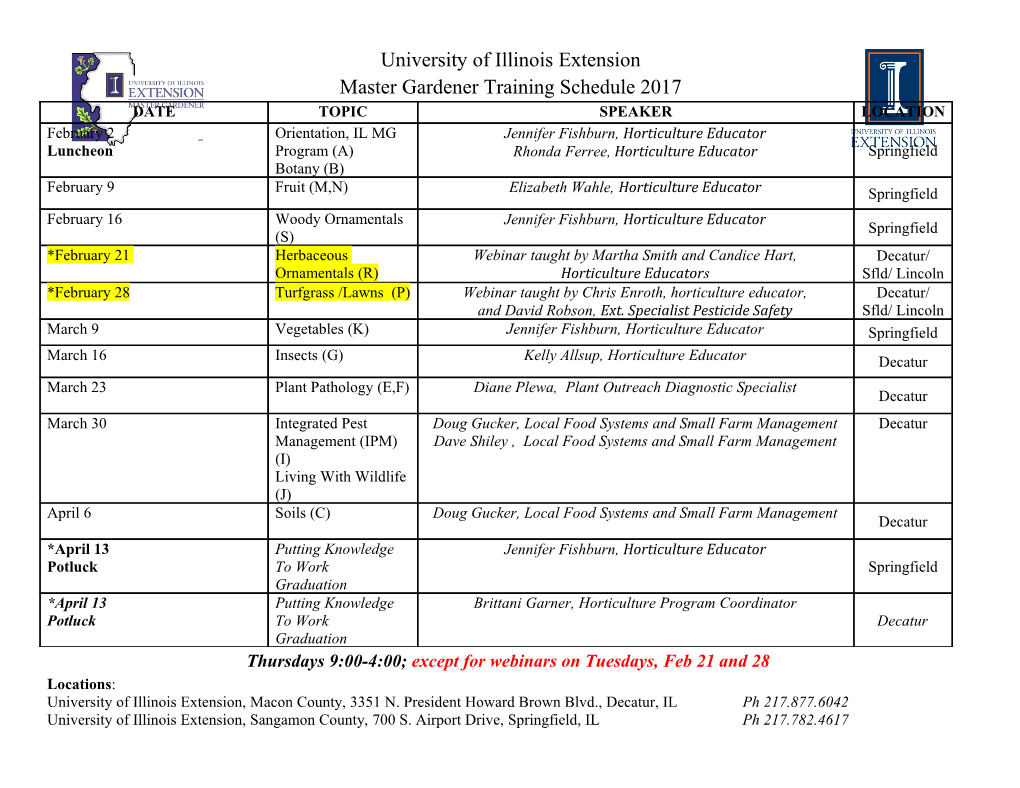
7. Cratering on Mars Impact cratering is one of the fundamen- 1987; Holsapple and Schmidt, 1987; Schmidt tal geological processes, and is visible on ev- and Housen, 1987; Croft, 1985b,a; O’Keefe and ery solid surface body in the solar system (e.g. Ahrens, 1981). The cratering process is con- Alfred Wegener, 1921). When the disrupted trolled by the target strength and for larger im- comet Shoemaker–Levi–9 hit Jupiter, it was pacting bodies by the surface gravity (Schmidt the only and the most spectacular opportu- and Housen, 1987). The final crater diameter nity to observe an impact event, but it left and appearance depends on gravity and mate- just short–term turbulences in the Jovian atmo- rial parameters (Croft, 1985b,a). Besides the sphere. On solid surfaces the impacting projec- knowledge of impact mechanism, other obvi- tiles form bowl–shaped depressions, which are ous differences in the impact environment in- named craters after a certain type of ancient fluences the final crater form, i.e. surface grav- Greek vase. Depending on the impacting en- ity, impact velocity, the presence of atmosphere ergy the crater morphology changes with diam- and the probable abundance of water or ice in eter. This progression was already described by the Martian subsurface. Gilbert in 1893. Mariner 9 images have revealed a diverse 7.1. Cratering Mechanics range of morphology and ejecta characteris- tics and potential target differences (Arvidson Independent of the final crater size, the process et al., 1976; McCauley, 1973). Impact crater of impact cratering always follows a three stage morphology and especially ejecta are largely scheme, which has been described in detail by unique to Mars (although they are found on Melosh (1989). The three stages of the impact the jovian moon Europa and on Earth). They cratering process are (1) contact and compres- have probably been strongly influenced by sub- sion, (2) excavation, and (3) modification (Fig. surface volatiles and are widespread through- 7.1). out the surface of the planet. The Martian im- During the first stage, the projectile contacts pact record in terms of crater morphology, mor- the target. As it hits, the target is instan- phometry, modification and crater frequency taneously compressed and accelerated, while has been catalogued by Strom et al. (1992), but the projectile itself is decelerated by the resis- their study also revealed the difficulties in the tance of the target. A shock wave originates at fundamental morphologic classification. Keys the point of contact and travels through target to explaining the large variety of central crater and projectile, producing pressures much larger structures or ejecta blankets include the geo- than the yield strength of either the target or logical conditions of the surface and subsurface the projectile. This stage, lasting a ”blink of an (possibly the atmosphere as well). The depen- eye”, ends after the shock wave has passed. dence of crater diameter on the condition of im- The second stage is divided into the expan- pacts, defined by the impacting body (radius, sion of the shock wave and the excavation flow. mass, density, impact velocity) and the plane- While the shock wave expands hemispherically tary surface (surface gravity, density, strength) (and subsequently degrades to a stress wave), is generally estimated by a form of scaling that the target material is set into motion radially employs both theory and small scale measure- away from the impact site (immediately behind ments to extrapolate to the large scales of ob- the shock wave). Rarefaction waves create an served craters and impact basins (Holsapple, upward–directed pressure gradient behind the 31 32 Cratering on Mars shock wave. This additional upward compo- nent to the radial velocity initializes the exca- vation flow and the largest portion of material ejects from the crater. This ejected material follows purely ballistic trajectories, the ejecta curtain. Material ejected first, closest to the impact site, has the highest velocities, and con- sequently takes the longest to fall. The growth of a crater up to the transient cavity starts as a hemispherical expansion following the shock wave until the final depth is achieved. Due to less resistance at the surface the crater diameter continues to grow further until its final diameter is achieved (Fig. 7.1). This state of the crater is called transient cavity. The final observed rim– to–rim diameter is different from the transient diameter and is reached in the last, so–called modification stage. During the final modification stage, the ejected debris is finally deposited and the crater interior becomes modified. The fundamental Figure 7.1.: The growth of a crater: The crater idea of ejecta emplacement is that the ejected first opens following the hemispherical expansion of material travels in a near parabolic trajec- the shock wave (a). The growth rate is steadily tory as a so–called ejecta curtain (ballistically). decreasing due to the resistance of the underlying Based on observations, one expects very lit- target rocks until its maximum depth is achieved tle material to escape the gravitational field (b). Less resistance at surface let the crater di- of a planet, most falling back to the surface ameter continue to grow (c) and stops when no and forming a continuous ejecta blanket sur- more material is ejected. The resulting transient crater is broader than a hemisphere (d) (Fig. from rounding the crater. The innermost ejecta are Melosh (1989)). Depending of the size of the tran- launched first, travel fastest and can reach long sient crater, modification in the given gravity field distances. Ejecta originating farther from the results in different morphologies. center are launched later, moving more slowly and falling sooner and closer to the rim. Only material from the uppermost third to half of the depth of the transient crater is excavated can be found, which are formed by large chunks from the crater. Target material deeper than of material during the excavation period. On the maximum excavation depth has been dis- the Moon, secondary craters can reach a size placed beneath the crater floor. The maximum of about 4% the diameter of the primary im- excavation depth (and the strata represented pact crater. The secondary crater itself is not in the ejecta blanket) is considerably shallower necessarily distinguishable on the basis of its than the maximum crater depth. Close to the morphology (they could appear more elliptical), rim, material is deposited in reversed origi- but ejecta of secondaries have a characteristic nal stratigraphy (overturned flap, Roddy et al. V–shaped ridge pointing radially away from the (1975)). The continuous ejecta blanket covers main crater. Cluster or chain formation also an area of roughly one crater radius from the help to identify them. Lined up as a chain, the crater rim, followed by a thin and patchy unit. chevron pattern turns into a herringbone pat- In the latter unit and beyond, secondary craters tern (Oberbeck and Morrison, 1973). In gen- Impact Crater Morphologies 33 eral, these crater–field forms are all strongly behavior of the impact cratering event. These controlled by the gravity field of the planet. experiments are limited in reproducing the ef- The change of the crater interior during the fects of high–energy impacts, which involve ex- modification stage varies, depending upon the treme pressures, temperatures and large crater– size of the transient cavity, and the shape char- diameter range (role of gravity during the for- acteristic of simple or progressively complex im- mation of large craters). Therefore, computer pact craters. For small craters, forming a final simulations are the only feasible method to simple bowl–shaped crater, the transient cav- study large–scale impact events. The complex- ity is filled by material falling back from the ity of the process involved during the crater for- unstable rim to subsequently fill the center of mation, especially the passage of shock waves the crater (wall slumping, Moore (1976)). The and the irreversible behavior of geologic ma- larger an impact crater, the further the ini- terial are translated into computer codes that tial transient departs from gravitational stabil- handle the shock wave propagation (velocities, ity and is modified more strongly during this stresses and strains) as a function of time and last crater forming stage. position. These are called hydrodynamical computer codes (”hydrocodes”). These codes 7.2. Crater Modeling are based on a discretized particle motion es- tablished through the principles of conserva- Currently, the applied crater size–frequency tion of momentum, mass and energy from a distribution is based on the transferred lunar macroscopic point of view (Anderson, 1987), curve. This transfer procedure is outlined in the equation–of–state, relating pressure, den- Section 5 and discussed in detail by Neukum sity and the specific internal energy (needed and Ivanov (1994); Ivanov (2001). To fur- to describe compressibility effects and irre- ther refine and verify the modeling and trans- versible thermodynamic processes), and a rhe- fer of the Martian crater production function, ology (constitutive) model, which describes the pre–existing modeling efforts have been contin- response of a material to deformation (change ued by refining existing scaling laws, improv- in shape or strength properties). ing models for crater collapse, and estimat- One of the most important issues concerning ing the impacting flux of planetesimals, includ- hydrocodes is the description of the discontin- ing comets (Ivanov, 2001). Target parameters uous nature of shock waves, which may intro- such as subsurface water, sedimentary and vol- duce instabilities in the discretized representa- canic rocks of different water or ice saturation tion. The choice of a discretization approach stages lead to a variety of scenarios that in- and solving methods depends on the problem fluence the final crater diameter, and there- to be solved, so a variety of hydrocodes have fore deviate from the analytical Martian crater been developed.
Details
-
File Typepdf
-
Upload Time-
-
Content LanguagesEnglish
-
Upload UserAnonymous/Not logged-in
-
File Pages10 Page
-
File Size-