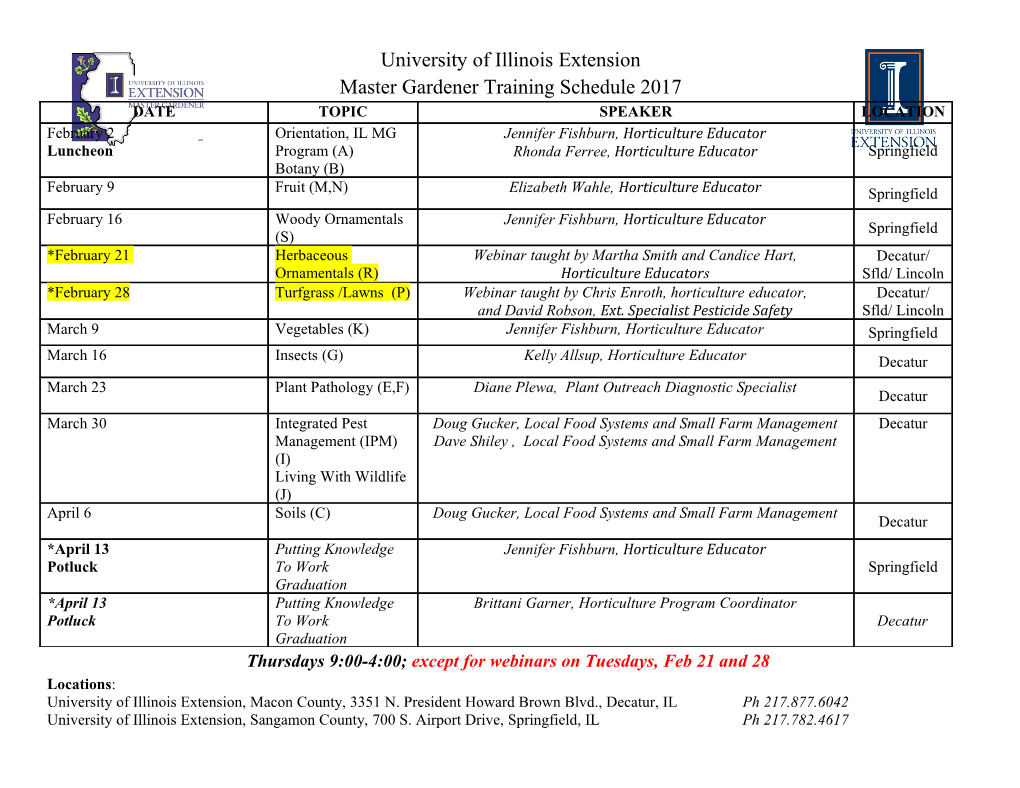
University of Nevada, Reno Home range, spatial dynamics, and growth of Moapa dace (Moapa coriacea) A thesis submitted in partial fulfillment of the requirements for the degree of Master of Science in Biology by Mark E. Hereford Dr. Mary M. Peacock/Thesis Advisor May, 2014 © by Mark E. Hereford 2014 All Rights Reserved THE GRADUATE SCHOOL We recommend that the thesis prepared under our supervision by MARK EARL HEREFORD entitled Home range, spatial dynamics, and growth of Moapa dace (Moapa coriacea) be accepted in partial fulfillment of the requirements for the degree of MASTER OF SCIENCE Mary M. Peacock, Ph.D., Advisor Chris R. Feldman, Ph.D., Committee Member Jill S. Heaton, Ph.D., Graduate School Representative David W. Zeh, Ph.D., Dean, Graduate School i ABSTRACT Moapa dace (Moapa coriacea) is an endangered thermophilic minnow (Family: Cyprinidae) native to the upper Muddy River which originates at geothermal springs in southeastern Nevada, USA. Historically Moapa dace occupied tributaries and the main stem of the Muddy River totaling 18 kilometers of stream habitat near the geothermal sources where water temperatures are between 26.0°C and 32.0°C. Due to habitat fragmentation, water diversion, and invasive species introductions during the early and mid-1900’s Moapa dace populations drastically declined. In 1997, following the invasion of the non-native blue tilapia (Oreochromis aureus), a fish barrier was installed isolating three tributaries from the main stem of the Muddy River for the protection of native aquatic organisms above the barrier. During this study Moapa dace were restricted to stream habitat above this barrier totaling 2.8 kilometers. The goals of this study were to determine the home range size, spatial dynamics, and growth of Moapa dace in these three tributaries of the Muddy River. A mark-recapture method using baited minnow traps was implemented and genetic tagging of individuals based on their unique genotypes using ten polymorphic microsatellites was used to identify individuals over a three year period (October 2009 – September 2012). Using geographic information systems (GIS) I was able to demonstrate that home range density (number of individual home ranges per square meter) was most restricted in a fragmented tributary and home range density increased in a tributary immediately following stream habitat restoration. Mean home range length of individuals increased in the last year of this study (141.3 meters), was greatest in the largest tributary (215.2 meters), and was the least in the ii fragmented tributary (70.5 meters). Growth rates of Moapa dace drastically decline in individuals over 40 mm (fork length), are highest between May and July, and lowest between September and November. Using Fulton’s body condition factor (K=W/L3) two distinct periods of low (January – March) and high (May – November) body condition were discovered. Growth rates and body condition were both significantly higher in individuals with home ranges over 25 meters. The results of this study suggest that Moapa dace will immediately occupy stream habitat that has been recently restored to increase stream velocities and re-emphasizes the importance of habitat connectivity as it relates to an individual’s ability to maximize energy intake. iii ACKNOWLEDGEMENTS This thesis would not have possible without the assistance of many individuals. I would like to thank Gary Scoppettone for his guidance and mentorship throughout this and many projects I have worked on, his teachings of his extensive knowledge of native fishes in western North America is something I am very grateful for. I would also like to thank my major advisor Dr. Mary Peacock whose encouragement, determination, and good spirits allowed me to complete this endeavor. The Southern Nevada Public Land Management Act, United States Fish and Wildlife Service, and the United States Geological Survey provided funding and logistical support for this project. Special thanks to Lee Simons and Amy LaVoie of the United States Fish and Wildlife Service and David Syzdek and staff of the Southern Nevada Water Authority for field logistical support. Without the hard work of Mark Fabes and Antonio Salgado of the United States Geological Survey this project could not have been completed. I would also like to thank Veronca Kirchoff for her knowledge and patience in teachings of molecular laboratory techniques which allowed me to analyze many samples. Thank you to my family and friends who have supported me throughout this process. Last but not least I would like to thank my lovely, brainy, and buff wife, Danielle Hereford, who not only worked with me throughout every aspect of field, laboratory, and analysis of this project but whose encouragement and enthusiasm allowed me to complete this project in a timely manner while still enjoying every process along the way. iv TABLE OF CONTENTS Page Introduction 1 Methods 8 Results 21 Discussion 26 Management/Conservation Implications 31 Literature Cited 36 v LIST OF TABLES Page Table Legends 45 Table 1. Summary of in-stream habitat characteristics 46 Table 2. Moapa dace microsatellite primer information 47 Table 3. Summary of fishes captured 48 vi LIST OF FIGURES Page Figure Legends 49 Figure 1. Origin of the Muddy River, Nevada 51 Figure 2. Warm Springs area of the Muddy River, Nevada 52 Figure 3. Sampling locations within the Warm Springs area 53 Figure 4. Average monthly water temperatures within study area 54 Figure 5. Home range density of Moapa dace 55 Figure 6. Mean home range length of Moapa dace among years 56 Figure 7. Mean home range length of Moapa dace among tributaries 57 Figure 8. Relationship of home range length and distance downstream 58 Figure 9. Growth rates of different Moapa dace size classes 59 Figure 10. Seasonal growth rates of Moapa dace 60 Figure 11. Monthly body condition of Moapa dace 61 1 INTRODUCTION Surface water habitat only makes up 0.01% of Earth’s total water and only 0.8% of Earth’s surface but supports more than 100,000 aquatic species, or 6% of all described species (Dudgeon et al. 2006). Almost half of the described vertebrate species on Earth are fishes with 700 fish species found in the freshwater ecosystems of North America (Allen and Flecker 1993; Helfman et al. 2009). Even with this extraordinary amount of diversity freshwater fishes and their ecosystems are one of the most threatened in the world (Ward 1998; Malmqvist and Rundle 2002; Dudgeon et al. 2006). There are many causes for the decline in biodiversity of freshwater fishes, including: habitat loss and degradation, habitat fragmentation, invasive species, overexploitation, secondary extinctions, chemical and organic pollution, and climate change (Allen and Flecker 1993; Fahrig 2003). Invasive non-native fish compete within trophic level, hybridize with native fish, use spawning habitat of native fishes, and can directly consume native fishes (Allen and Flecker 1993; Rahel 2000; Poff et al. 2007). Habitat fragmentation in lotic ecosystems can have an adverse effect on the amount of genetic diversity that is maintained within fishes. Not only does fragmentation disrupt migratory fish from reaching their spawning grounds and disrupt metapopulation dynamics among populations (Allen and Flecker 1993; Willson and Halupka 1995), but it can also lead to a loss of genetic diversity in populations due to isolation, smaller habitats, and lower recruitment (Neilsen et al. 1997; Alo and Turner 2005). Freshwater fishes living in desert aquatic ecosystems are one of the most threatened groups of organisms. Of all the fish species on the United States Endangered 2 Species List, the majority live in desert aquatic environments (Ono et al. 1983). Desert fishes are typically restricted to small, isolated areas with limited amounts of surface water which originate from springs supplied by water from aquifers. Due to increasing human populations in the southwest deserts of North America water demand for agriculture, industrial, and consumptive purposes have greatly increased habitat loss and fragmentation, as well as the introduction of non-native species into these unique and isolated desert aquatic ecosystems (Ono et al. 1983; Williams et al. 1985). The Moapa dace (Moapa coriacea), the only species of the Moapa genus, is a thermophilic cyprinid endemic to the headwaters of the Muddy River, Clark County, in southern Nevada. It was first collected and described in 1938 in the upper reaches of the Muddy River, (Hubbs and Miller 1948). Historically, Moapa dace has had an incredibly restricted distribution of around 16 kilometers of stream habitat in the main stem of the upper Muddy River and its many tributaries, which originate as geothermal springs (Ono et al. 1983). When first discovered by Hubbs and Miller (1948) Moapa dace were abundant but only inhabited the upper reaches of the Muddy River and its geothermal tributaries (i.e. Warm Springs area), in water varying from 26 to 32 degrees Celsius along with another native thermophilic fish, the Moapa White River springfish (Crenichthys baileyi moapae). The Muddy River originates from more than 20 springs within a two kilometer radius in the Warm Springs area of the Moapa Valley in the Mojave Desert (Scoppettone et al. 1998) and contains 28 at-risk or rare species including ten rare and one endangered endemic aquatic species (USFWS 1995). The Muddy River is one of the remnants of 3 Pluvial White River, which drained the majority of southeastern Nevada during the late Pleistocene Epoch (Wisconsin Glacial Episode, 85,000 – 11,000 years ago) (Hubbs and Miller 1948). Before the construction of the Hoover dam on the Colorado River and the creation of Lake Mead in 1936, the Muddy River was a tributary to the Virgin River which then joined the Colorado River (Hubbs and Miller 1948). Currently the Muddy River flows into the Overton Arm of Lake Mead. Because the Muddy River system is a rare oasis in the Mojave Desert, the river has been greatly altered by humans.
Details
-
File Typepdf
-
Upload Time-
-
Content LanguagesEnglish
-
Upload UserAnonymous/Not logged-in
-
File Pages70 Page
-
File Size-