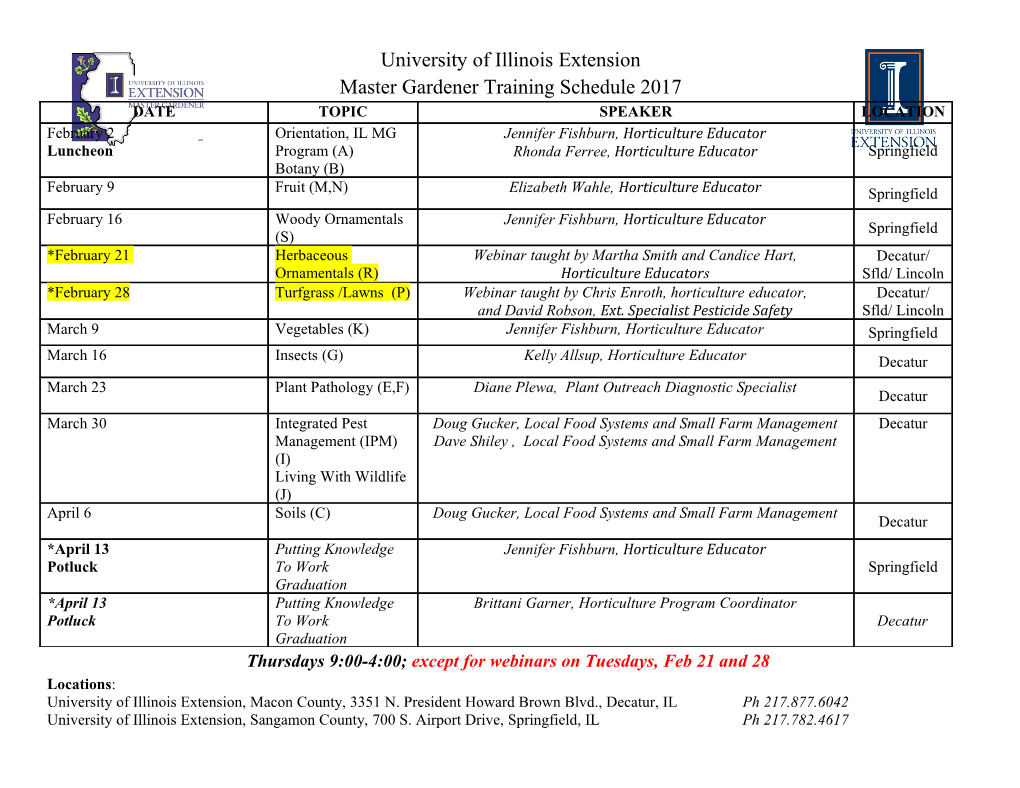
Mathematics and Statistics 9(2): 179-187, 2021 http://www.hrpub.org DOI: 10.13189/ms.2021.090213 A Dirac Delta Operator Juan Carlos Ferrando Centro de Investigacion Operativa, Universidad Miguel Hernandez, E-03202 Elche, Spain Received February 1, 2021; Revised March 18, 2021; Accepted April 9, 2021 Cite This Paper in the following Citation Styles (a): [1] Juan Carlos Ferrando, ”A Dirac Delta Operator,” Mathematics and Statistics, Vol.9, No.2, pp. 179-187, 2021. DOI: 10.13189/ms.2021.090213. (b): Juan Carlos Ferrando, (2021). A Dirac Delta Operator. Mathematics and Statistics, 9(2), 179-187. DOI: 10.13189/ms.2021.090213. Copyright ©2021 by authors, all rights reserved. Authors agree that this article remains permanently open access under the terms of the Creative Commons Attribution License 4.0 International License Abstract If T is a (densely defined) self-adjoint opera- operator λ 7! δ (λI − T ) at T by tor acting on a complex Hilbert space H and I stands for Z +1 the identity operator, we introduce the delta function opera- f (T ) = f (λ) δ (λI − T ) dλ (1.1) tor λ 7! δ (λI − T ) at T . When T is a bounded operator, −∞ then δ (λI − T ) is an operator-valued distribution. If T is un- bounded, δ (λI − T ) is a more general object that still retains for each f 2 C (R), i. e., for each real-valued continuous func- some properties of distributions. We provide an explicit repre- tion f (λ). Here dλ is the Lebesgue measure of R, but the sentation of δ (λI − T ) in some particular cases, derive vari- right-hand side of (1.1) is not a true integral. If T is a bounded ous operative formulas involving δ (λI − T ) and give several operator, we shall see at once that δ (λI − T ) must be regarded applications of its usage in Spectral Theory as well as in Quan- as a vector-valued distribution, i. e., as a continuous linear map tum Mechanics. from the space D (R) into the locally convex space L (H) of Keywords Hilbert Space, Self-adjoint Operator, Vector- the bounded linear operators (endomorphisms) on H equipped valued Distribution, Spectral Measure with the strong operator topology [10, 11], whose action on f 2 D (R) we denote as an integral. If T is unbounded we shall see that δ (λI − T ) still retains some useful distributional-like properties. The previous equation means Z +1 δ (λI − T ) hy; f (T ) xi = f (λ) hy; δ (λI − T ) xi dλ (1.2) 1 The delta function −∞ The scalar delta ‘function’ λ 7! δ (λ − a) along with its for each (x; y) 2 D (f (T )) × H, where D (f (T )) stands for derivatives were introduced by Paul Dirac in [1], and later in the domain of the self-adjoint operator f (T ). [2, Section 15], although its definition can be traced back to Let us recall that if T is a (densely defined) self-adjoint op- Heaviside. The rigorous treatment of this object in the context erator, there is a unique spectral family fEλ : λ 2 Rg of self- of distribution theory is due to Laurent Schwartz [6, 12]. In this adjoint operators defined on the whole of H that satisfy (i) paper we extend the definition of δ (λ − a) from real numbers Eλ ≤ Eµ and EλEµ = Eλ for λ ≤ µ, (ii) lim!0+ Eλ+x = to self-adjoint operators on a Hilbert space H. We denote by Eλx, and (iii) limλ→−∞ Eλx = 0 and limλ!1 Eλx = x in H x 2 H D (T ) T D (R) =−! lim D ([−n; n]) the linear space of infinitely differen- for all . The domain of consists of those tiable complex-valued functions of compact support, equipped x 2 H such that with the inductive limit topology. As usual in physics we shall Z +1 2 2 assume that the scalar product in H is anti-linear for the first jλj d kEλxk < 1: variable. −∞ If T is a densely defined self-adjoint operator1 on H and I In this case, the spectral theorem (cf. [8, Section 107]) and stands for the identity operator, we define the delta function the Borel-measurable functional calculus provide a self-adjoint operator f (T ) defined by 1In what follows σ (T ) will denote the spectrum of T . Recall that the residual spectrum of a self-adjoint operator T is empty, so that σ (T ) = Z +1 σp (T ) [ σc (T ), where σp (T ) denotes the point spectrum (the eigenvalues) f (T ) = f (λ) dE (1.3) and σ (T ) the continuous spectrum of T . λ c −∞ 180 A Dirac Delta Operator for each Borel-measurable function f (λ), whose domain If λ 7! Y (λ − µ) denotes the unit step function at µ 2 R, given by Y (λ − µ) = 0 if λ < µ and Y (λ − µ) = 1 if λ ≥ µ, Z +1 2 2 since Eλ = Y (λI − T ) for each λ 2 R, formally D (f (T )) = x 2 H : jf (λ)j d kEλxk < 1 −∞ 0 dEλ/dλ = Y (λI − T ) : (1.7) is dense in H. Observe that if T is bounded, f (T ) need not So, from (1.6) and (1.7) we get Y 0 (λI − T ) = δ (λI − T ). be bounded. Moreover, since λ 7! Eλ is constant on the set R n σ (T ) of T , an open set in R, equation (1.3) tell us that Proposition 1. If T is a bounded self-adjoint operator on H f (λ) need not be defined on n σ (T ). 1 R and f 2 C (R), then Thanks to (1.3) the definition of δ (λI − T ) may be extended Z +1 to Borel-measurable functions by declaring that the equation 0 0 (1.1) holds for (x; y) 2 D (f (T )) × H and each Borel func- f (λ) δ (λI − T ) dλ = −f (T ) : −∞ tion f. But, by reasons that will become clear later, we shall restrict ourselves to those Borel functions which are continuous The same equality holds if T is unbounded but f 2 D (R). at each point of σp (T ). Moreover, working with the real and complex parts, no difficulty arises if the function f involved If T is a self-adjoint operator and f 2 Bp (R), then in the equation (1.1) is complex-valued (except that f (T ) is Z + 1 Z + 1 2 2 no longer a self-adjoint operator whenever Imf 6= 0). Thus, jf (λ)j δ (λI − T ) dλ = jf (λ)j dEλ: unless otherwise stated, we shall assume that both in (1.1) and −∞ −∞ (1.3) the function f is complex-valued. Note that the complex where the latter equality is the definition of jf (T )j2. So, we Stieltjes measure d hEλx; yi need not be dλ-continuous. In have the following result. what follows we shall denote by Bp (R) the linear space over C consisting of all complex-valued Borel-measurable functions Proposition 2. If T is self-adjoint and f 2 Bp (R), then of one real variable which are continuous on σp (T ). 1 + 1 If fn ! f in D (R), the sequence ffngn=1 is uniformly Z hf (T ) y; f (T ) xi = jf (λ)j2 hy; δ (λI − T ) xi dλ bounded and fn (x) ! f (x) at each x 2 . So, if T is R −∞ bounded on H (equivalently, self-adjoint on the whole of H) it turns out that fn (T ) ! f (T ) in the strong operator topol- for every x; y 2 D (f (T )). ogy [3, 10.2.8 Corollary]. Therefore, in this case δ (λI − T ) is an L (H)-valued distribution. Proof. We adapt a classic argument. Indeed, for every x; y 2 As all integrals considered so far are over σ (T ), we have D (f (T )) we have Z + 1 δ (λI − T ) = 0 8λ2 = σ (T ) : (1.4) hf (T ) y; f (T ) xi = f (λ) d hf (T ) y; Eλxi : −∞ Also δ (−λI + T ) = δ (λI − T ) for all λ 2 . On the other R E E = E µ ≤ λ hE y; xi hand, if µ 2 σ (T ) and y is an eigenvector corresponding to Since µ λ µ whenever , and λ does not p µ the eigenvalue µ, clearly depend on , by splitting the integral we get Z + 1 Z λ δ (λI − T ) y = δ (λ − µ) y (1.5) f (µ) d hEµy; Eλxi = f (µ) d hy; Eµxi ; (1.8) −∞ −∞ for every λ 2 R. In the particular case when Ta is the linear where clearly the first integral is hf (T ) y; Eλxi. Plugging operator defined on H by Tax = ax for a fixed a 2 R, then Ta d hf (T ) y; Eλxi into (1.8), we are done. is a self-adjoint linear operator with σ (Ta) = σp (Ta) = fag. In this case δ (λI − Ta) x = δ (λ − a) x for every x 2 H, i. e., Corollary 3. Under the same conditions of the previous theo- δ (λI − Ta) = δ (λ − a) I. rem, the equality D E Since equality f (T )y y; x = hy; f (T ) xi holds for all Z + 1 2 2 x; y 2 D (f (T )) and each f 2 Bp (R), we may infer that kf (T ) xk = jf (λ)j hx; δ (λI − T ) xi dλ (1.9) −∞ hy; δ (λI − T ) xi = hδ (λI − T ) y; xi holds for every x 2 D (f (T )).
Details
-
File Typepdf
-
Upload Time-
-
Content LanguagesEnglish
-
Upload UserAnonymous/Not logged-in
-
File Pages9 Page
-
File Size-