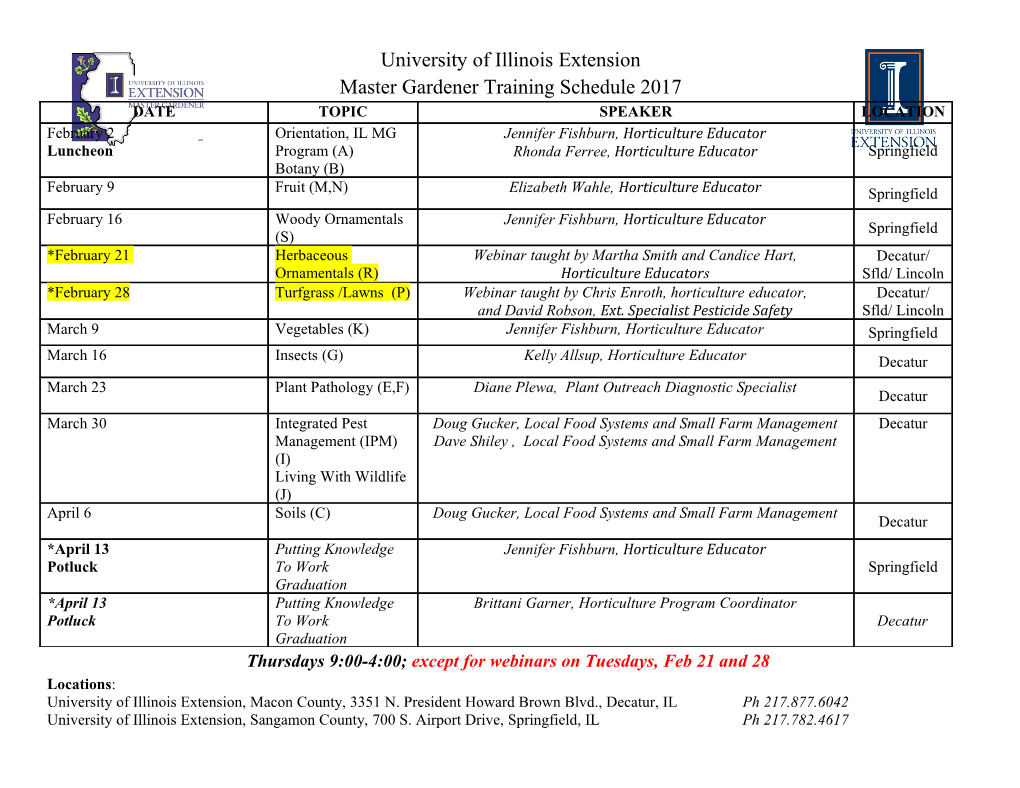
Received: 23 May 2019 Revised: 20 November 2019 Accepted: 30 November 2019 DOI: 10.1002/jmor.21088 RESEARCH ARTICLE Diversity of extracellular matrix morphology in vertebrate skeletal muscle David A. Sleboda1 | Kristin K. Stover1 | Thomas J. Roberts1 1Department of Ecology and Evolutionary Biology, Brown University, Providence, Rhode Abstract Island Existing data suggest the extracellular matrix (ECM) of vertebrate skeletal muscle con- Correspondence sists of several morphologically distinct layers: an endomysium, perimysium, and epi- *David A. Sleboda, Department of Ecology and mysium surrounding muscle fibers, fascicles, and whole muscles, respectively. These Evolutionary Biology, Brown University, Providence, RI. ECM layers are hypothesized to serve important functional roles within muscle, Email: [email protected] influencing passive mechanics, providing avenues for force transmission, and influenc- Present address ing dynamic shape changes during contraction. The morphology of the skeletal muscle Kristin K. Stover, Department of Biomedical ECM is well described in mammals and birds; however, ECM morphology in other ver- Science, West Virginia School of Osteopathic Medicine, Lewisburg, WV tebrate groups including amphibians, fish, and reptiles remains largely unexamined. It remains unclear whether a multilayered ECM is a common feature of vertebrate skele- Funding information Bushnell Research Fund; Drollinger Family tal muscle, and whether functional roles attributed to the ECM should be considered in Charitable Foundation; National Institute of mechanical analyses of non-mammalian and non-avian muscle. To explore the preva- Arthritis and Musculoskeletal and Skin Diseases, Grant/Award Number: AR55295; lence of a multilayered ECM, we used a cell maceration and scanning electron micros- National Institutes of Health, Grant/Award copy technique to visualize the organization of ECM collagen in muscle from six Number: S10 OD023461; National Science Foundation, Grant/Award Numbers: 1832795, vertebrates: bullfrogs (Lithobates catesbeianus), turkeys (Meleagris gallopavo), alligators IOS1354289 (Alligator mississippiensis), cane toads (Rhinella marina), laboratory mice (Mus musculus), and carp (Cyprinus carpio). All muscles studied contained a collagen-reinforced ECM with multiple morphologically distinct layers. An endomysium surrounding muscle fibers was apparent in all samples. A perimysium surrounding groups of muscle fibers was apparent in all but carp epaxial muscle; a muscle anatomically, functionally, and phylogenetically distinct from the others studied. An epimysium was apparent in all samples taken at the muscle periphery. These findings show that a multilayered ECM is a common feature of vertebrate muscle and suggest that a functionally relevant ECM should be considered in mechanical models of vertebrate muscle generally. It remains unclear whether cross-species variations in ECM architecture are the result of phylogenetic, anatomical, or functional differences, but understanding the influence of such variation on muscle mechanics may prove a fruitful area for future research. KEYWORDS collagen, connective tissue, fascia 1 | INTRODUCTION tissues frequently subjected to tensile forces, the ECM of skeletal muscle is reinforced by fibrous collagen (Borg & Caulfield, 1980). Col- Skeletal muscle contains a highly specialized extracellular matrix lagen has a close-packed triple helix structure that grants it a high (ECM) that suits its mechanical functions. Like skin, tendon, and other mechanical strength (Harkness, 1961), and its presence within muscle Journal of Morphology. 2019;1–10. wileyonlinelibrary.com/journal/jmor © 2019 Wiley Periodicals, Inc. 1 2 SLEBODA ET AL. influences many mechanical behaviors of the tissue. ECM collagen as the most likely vector through which forces are transmitted both influences the mechanical response of muscle to deformation, deter- laterally and serially between neighboring muscle fibers within the mining in part the passive tension developed by stretched muscle muscle belly (Purslow & Trotter, 1994). The perimysium contains rela- (Gindre, Takaza, Moerman, & Simms, 2013; Meyer & Lieber, 2011, tively larger collagen fibers (each composed of multiple collagen fibrils) 2018; Prado et al., 2005). The collagenous ECM also provides avenues which form fibrous septa that are shared by and delineate adjacent for lateral and serial transmission of force between adjacent muscle muscle fascicles (Borg & Caulfield, 1980; Purslow, 1989). Mathemati- fibers within the muscle belly (Huijing, Baan, & Rebel, 1998; Purslow & cal modeling suggests that the thickness of the perimysium renders it Trotter, 1994; Street, 1983), a function that allows coordination of an unlikely vector for transmission of force within muscle in vivo force from multiple muscle fibers within a fascicle, and which prevents (Purslow, 2010); however, an alternative role for the perimysium may interruptions to muscle function during tissue growth or repair be to provide slip planes within the muscle belly that accommodate (Purslow, 2010). The collagenous ECM also likely influences dynamic dynamic shape changes during contraction (Purslow, 1999; Purslow, changes in muscle shape during contraction, allowing the ECM to have 2002). The epimysium surrounds the muscle periphery and, like the a direct effect on the force and speed of contraction (Azizi, Brainerd, & perimysium, is composed of collagen fibers. Anatomical continuity Roberts, 2008; Eng, Azizi, & Roberts, 2018) as well as the useful between the epimysium, endomysium, perimysium, and in-series ten- mechanical work produced by a shortening muscle (Azizi, Deslauriers, dons suggest that the epimysium acts as a surface tendon, aiding in Holt, & Eaton, 2017). In cardiac muscle, ECM connections to vascula- the transmission of force from muscle fibers to the skeletal system in ture help maintain patency and prevent longitudinal slippage of vessels some muscles (Passerieux, Rossignol, Letellier, & Delage, 2007). during contraction (Caulfield & Borg, 1979) and the ECM may play a Predominant among morphological and functional studies of skel- similar protective role for vessels and nerves in skeletal muscle. etal muscle ECM are studies of mammalian and avian muscle. Mam- Existing data suggest the ECM of vertebrate skeletal muscle con- mals including cows, sheep, rats, rabbits, cats, pigs, and mice have sists of several morphologically distinct, hierarchically arranged layers been shown to display distinct endomysial, perimysial, and epimysial (Figure 1). These layers are the endomysium, perimysium, and epimy- ECM layers (see Table S1 for a list of studies of vertebrate skeletal sium, and they surround muscle fibers, fascicles, and whole muscles, muscle ECM morphology organized by species). Comparisons of ECM respectively (Borg & Caulfield, 1980; Gillies & Lieber, 2011; Rowe, morphology both across mammalian species (e.g., Rowe, 1981), and 1981). The endomysium consists of a network of criss-crossing colla- across anatomically distinct muscles within a single mammalian spe- gen fibrils that ensheathe and anchor on the cell membranes (sarco- cies (e.g., Borg & Caulfield, 1980), reveal that a collagenous, trilayered lemma) of muscle fibers. Endomysial collagen fibrils wrap the long ECM is typical of mammalian skeletal muscle. A similar morphological axes of individual muscle fibers, but are also shared between adjacent arrangement of the ECM has been observed in birds. Broiler chicken muscle fibers within a fascicle (Trotter & Purslow, 1992). They serve muscles display a collagenous ECM like that of mammals, with distinct endomysial, perimysial, and epimysial layers (e.g., Nakamura, Iwamoto, Tabata, & Ono, 2003; Roy et al., 2006). Although these studies show that a multilayered, collagen reinforced ECM is typical of mammals and birds, the morphological arrangement of collagen in the ECMs of other vertebrate groups including fish, amphibians, and reptiles has received relatively little study. Fibrous collagen has been shown to rein- force the ECMs of certain frogs (Schmalbruch, 1974) and fish (Ando, Toyohara, & Sakaguchi, 1992; Ando, Yoshimoto, Inabu, Nakagawa, & Makinodan, 1995), but whether these animals contain distinct endomysium, perimysium, and epimysium structurally similar to those described in mammals and birds is not clear from these studies. Without knowledge of ECM morphology in much of the verte- brate group, it cannot be stated with confidence that a multilayered ECM is a general feature of vertebrate skeletal muscle, or that func- tional roles attributed to the endomysium, perimysium, and epimy- sium should be considered in mechanical analyses of non-mammalian and non-avian muscle. Additionally, the efficacy of using non- mammalian or non-avian muscles as model systems for the study of ECM function remains untested. Amphibian muscle, for example, has been used as a model system to explore the influence of the ECM on FIGURE 1 Spatial organization of muscle fibers and extracellular lateral force transmission (Street, 1983) and passive tension devel- matrix as observed in mammalian and avian skeletal muscle. The extracellular matrix is a hierarchical, interlinked network composed of oped during stretch (Sleboda & Roberts, 2017; Sleboda, Wold, & Rob- distinct layers that surround muscle fibers (endomysium), fascicles erts, 2019). Knowledge of ECM morphology in these animals is (perimysium), and the muscle periphery (epimysium) needed to ensure the applicability of findings
Details
-
File Typepdf
-
Upload Time-
-
Content LanguagesEnglish
-
Upload UserAnonymous/Not logged-in
-
File Pages10 Page
-
File Size-