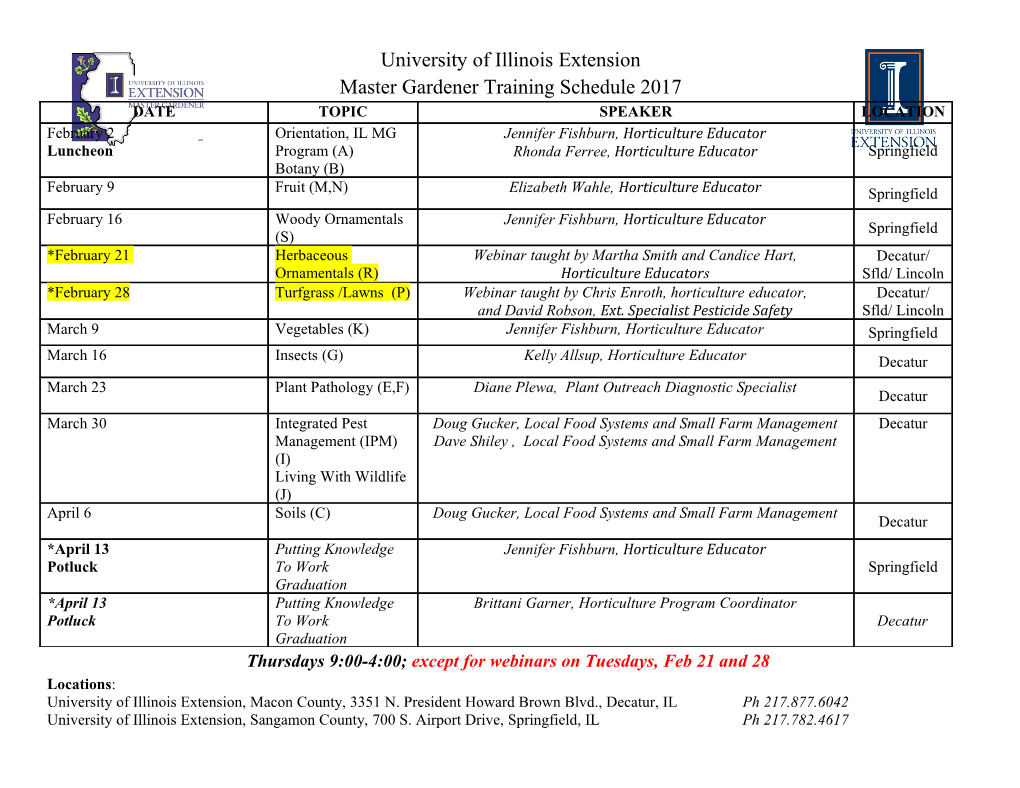
Health Science Campus FINAL APPROVAL OF DISSERTATION Doctor of Philosophy in Biomedical Sciences Pituitary Adenylate Cyclase Activating Polypeptide Signaling Alters Gene Expression In Chick Ciliary Ganglion Neurons Submitted by: Adriane D. Sumner In partial fulfillment of the requirements for the degree of Doctor of Philosophy in Biomedical Sciences Examination Committee Major Advisor: Joseph Margiotta, Ph.D. Academic Linda Dokas, Ph.D. Advisory Committee: David Giovannucci, Ph.D. Marthe Howard, Ph.D. Phyllis Pugh, Ph.D. Senior Associate Dean College of Graduate Studies Michael S. Bisesi, Ph.D. Date of Defense: January 22, 2008 Pituitary Adenylate Cyclase Activating Polypeptide Signaling Alters Gene Expression In Chick Ciliary Ganglion Neurons Adriane D. Sumner University of Toledo College of Medicine 2008 ACKNOWLEDGEMENTS I would like to thank my major advisor, Dr. Joseph Margiotta, and the other members of my advisory committee, Dr. Phyllis Pugh, Dr. Linda Dokas, Dr. Marthe Howard, and Dr. David Giovannucci, for their advice and support during my graduate education. I would like to thank the members of my lab; Dr. Gail Adams, whose help made the microarray experiments possible, Dr. Phyllis Pugh, who designed the primers used in the PAC1 splice variant and VPAC receptor conventional RT-PCR experiments, and Selwyn Jayakar, who has not only been a valuable colleague but a wonderful friend. I am very thankful for the love and support of my family and friends. I am especially grateful to my mom, Pat Sumner, and my sister, Erin Barthel, for all of those long talks and words of encouragement which sustained me through the tough times. I would never have made it without them. ii TABLE OF CONTENTS Acknowledgements ii Table of Contents iii Introduction 1 Literature 6 Methods and Materials 25 Results 38 Discussion 77 Conclusions 89 References 90 Abstract 108 iii INTRODUCTION Neuropeptides often co-localize to the same presynaptic terminals as conventional neurotransmitters and have been shown to modulate neurotransmitter functions as well as influence neuronal development and regulation (Hokfelt et al. 2000). The neuropeptide, PACAP (Pituitary adenylate cyclase-activating polypeptide), is known to be present throughout the central nervous system (CNS), the peripheral nervous system (PNS), and even in some peripheral organs (Peeters et al. 2000) and has been implicated in a wide range of biological functions (Vaudry et al. 2000). PACAP can bind to three types of G protein-coupled receptors (GPCRs); VPAC1, VPAC2, and PAC1. The VPAC1 and VPAC2 receptors have an equal affinity for both PACAP and its most closely related peptide, vasoactive intestinal peptide (VIP) which shares 68% sequence identity with PACAP. However, PAC1 is a specific PACAP receptor with a 100-1000 fold greater affinity for PACAP than for VIP (Vaudry et al. 2000). PAC1 is also known to have a number of splice variants, the majority of which result from inclusion or exclusion of exons in the N-terminal domain which determines ligand binding specificity and/or the third intracellular loop which determines the G-protein or ion channel to which the receptor will couple (Lutz et al. 2006). Previous studies from this laboratory have demonstrated that PACAP is present in the chick parasympathetic ciliary ganglion (CG) at developmental stages from embryonic day 8 (E8) through E20 (Margiotta and Pardi 1995; Pugh and Margiotta 2006). However, its location within the CG is unknown. While the presence of PAC1 receptors has also been established, it is still unknown if VPAC1 or VPAC2 are present in the chick CG. 1 This laboratory has also demonstrated that application of exogenous PACAP results in activation of PAC1 receptors leading to increased signaling through adenylate cyclase (AC) and phospholipase C (PLC), resulting in increased cAMP production and 2+ intracellular calcium (Ca in) respectively (Margiotta and Pardi 1995; Pardi and Margiotta 1999). In the chick CG, PACAP has been shown to have temporally diverse effects, exerting both gradual, survival-promoting trophic actions (Pugh and Margiotta 2006) and rapid-onset neuromodulatory effects impacting nicotinic receptors and synapses (Margiotta and Pardi 1995; Pardi and Margiotta 1999). Neuronal survival in the CG is influenced by preganglionic synaptic transmission, innervation of postganglionic targets, and neurotrophins (Nishi and Berg 1981a; Nishi and Berg 1981b). Most CG neurons do not survive when maintained in a basal culture medium lacking tissue extracts over a period of seven days (Landmesser and Pilar 1974a). However, this laboratory has demonstrated that the percentage of neurons that survive increases to approximately 70% when the culture medium is supplemented with exogenous PACAP (Pugh and Margiotta 2000). The fact that the concentration of PACAP levels off during the period of cell death in the CG suggest that PACAP plays an important role in neuronal survival in vivo as well. The neurotrophic properties of PACAP in the CG are dependent upon the AC signaling cascade involving both cAMP and PKA and by the mitogen-activated protein kinase (MAPK) signaling pathway which involves the phosphorylation of MAPK by MEK, but not the PLC signaling cascade (Pugh and Margiotta 2006). 2 The neuromodulatory actions of PACAP are rapid, occurring in most cases within minutes (Pardi and Margiotta 1999). In CG neurons, PACAP signaling rapidly influences the function of both -bungarotoxin- ( Bgt-) sensitive nicotinic acetylcholine receptors, containing only 7 subunits ( 7-nAChRs), and heteropentameric receptors, containing 3, 5, 4, and, in some cases, 2 subunits ( 3*-nAChRs) (Vernallis et al. 1993; Nai et al. 2003) which underlie normal ganglionic neurotransmission (Zhang et al. 1994; Wilson Horch and Sargent 1995). Specifically, after brief PACAP treatment (10- 60 min), both 7-nAChR and 3*-nAChR-mediated whole-cell currents recorded from CG neurons markedly increase, an effect requiring PAC1 receptors and a signal cascade triggering AC activation, cAMP accumulation, and PKA phosphorylation (Margiotta and Pardi 1995). The net nAChR modulation likely depends on an interplay between AC and PLC activation since after blocking AC with 2‟5‟-dideoxyadenosine (ddA), PACAP treatment rapidly leads to selective inhibition of 7-nAChR currents by a PLC-dependent 2+ process requiring inositol phosphate (IP) turnover and increased Ca in that leaves 3*- nAChR currents unaffected (Margiotta and Pardi 1995; Pardi and Margiotta 1999). Relevant to synaptic interactions, exposure to PACAP enhanced activity at synapses that form between CG neurons in culture, utilizing PAC1 -, AC-, cAMP- and PKA-dependent processes to increase both the frequency and amplitude of nAChR-mediated spontaneous excitatory synaptic currents (sEPSCs) by 400% and 50%, respectively (2004 Abstract and Pugh and Margiotta, unpublished). While these changes in synaptic function occur within minutes of PACAP application, they were sustained for up to 48 hours following a single 15 min exposure (Pugh and Margiotta, unpublished). These results demonstrate 3 that PACAP actions have long term consequences on both trophic and modulatory functions which could result from alterations in gene expression. This theory is further supported by the fact that changes in intracellular calcium, cAMP, and MAPK, which are observed in response to PACAP, have all previously been linked to the regulation of gene transcription as well (Squire et al. 2003). Therefore, in this study, the ability of PACAP to alter gene expression was explored. Immunostaining and microscopy techniques were used to examine the ability of PACAP to phosphorylate and activate the transcription factor CREB (cAMP/Ca2+ response element binding protein) and microarrays were used to investigate the global effect PACAP has on altering gene transcription. The activation of the transcription factor CREB was characterized as a marker for gene expression since CREB has previously been implicated in neuronal plasticity and survival and has been shown to be activated by increased levels of intracellular calcium, cAMP, and MAPK (Bito and Takemoto 2003). In this study, it is demonstrated that application of exogenous PACAP results in the phosphorylation of CREB at Ser133 in a time- and concentration-dependent manner, an effect that persists for at least 90 minutes after only a 15 min exposure to PACAP. The ability of PACAP to activate CREB requires PAC1 since it was mimicked by the PAC1 selective agonist, maxadilan, inhibited by the PAC1 antagonist, PACAP(6- 38), and showed an expected increase in the EC50 of the concentration curve when induced by VIP. PKA may play a role in the intracellular signaling cascade since two pharmacological blockers of PKA, H89 and KT5720, inhibited PACAPs ability to phosphorylate CREB. 4 It was also examined whether varying PACAP exposure regimens result in recruitment of unique or overlapping sets of genes. Using the Affymetrix (Affymetrix inc., Santa Clara, CA) 28K chicken genome array it was demonstrated that brief (15min), intermediate (24h), and chronic (96h) treatment with exogenous PACAP differentially alters gene expression in E8 CG neurons maintained in cell culture for four days. Analysis with Genesifter revealed 672 known genes regulated by PACAP including genes implicated in synaptic function, neuronal growth, survival, and development. Each PACAP treatment condition altered a unique set of genes. However, 9 genes were up- regulated and 2 genes were down-regulated in all three PACAP treatment
Details
-
File Typepdf
-
Upload Time-
-
Content LanguagesEnglish
-
Upload UserAnonymous/Not logged-in
-
File Pages113 Page
-
File Size-