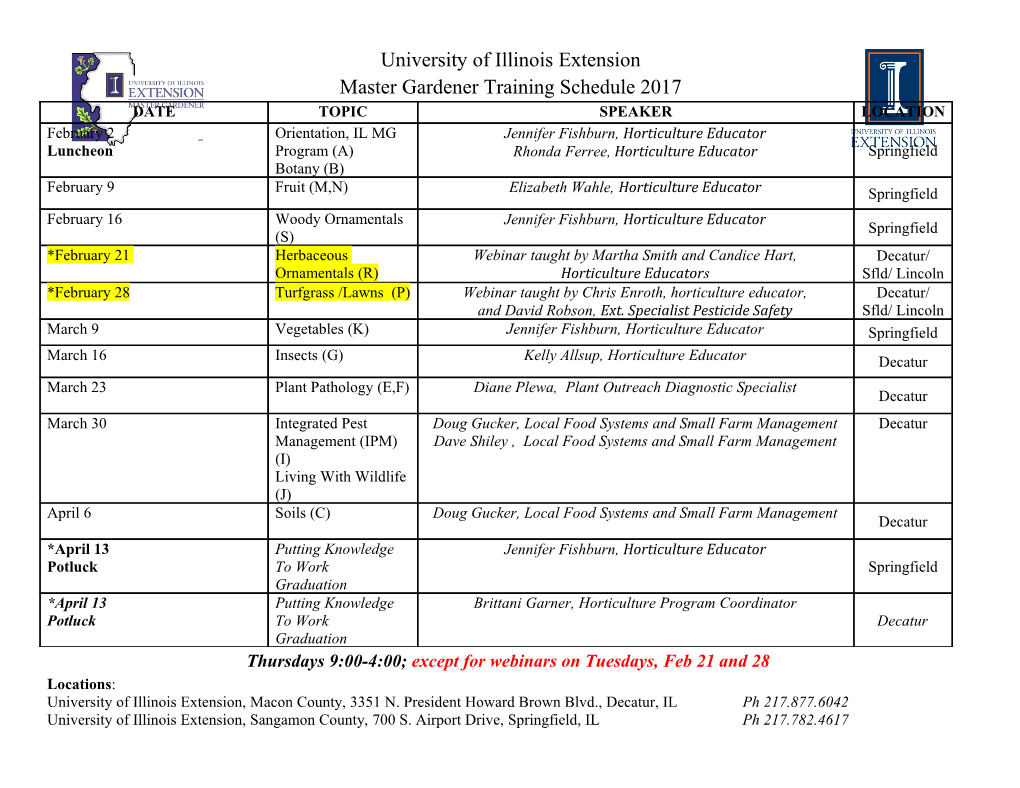
Journal of Structural Geology 24 72002) 1179±1193 www.elsevier.com/locate/jstrugeo Dissolution and replacement creep: a signi®cant deformation mechanism in mid-crustal rocks R.P. Wintscha,*, Keewook Yia,b aDepartment of Geological Sciences, Indiana University, Bloomington, IN 47405, USA bSchool of Earth and Environmental Sciences, Seoul National University, Seoul, South Korea Received 4 December 2000; revised 2 June 2001; accepted 26 June 2001 Abstract Zoning patterns and zoning truncations in metamorphic minerals in a granodioritic orthogneiss indicate that strain and S±C fabrics in these rocks were produced by dissolution, precipitation, and replacement processes, even at epidote±amphibolite facies metamorphic conditions. The metamorphic fabric is de®ned by alternating layers and folia dominated by quartz, feldspars, and biotite 1 epidote. Zoning patterns in most metamorphic plagioclase, orthoclase, epidote, and sphene are truncated at boundaries normal to the shortening direction, suggesting dissolution. Interfaces of relict igneous orthoclase phenocrysts that face the shortening direction are embayed and replaced by biotite, epidote, and myrmekitic intergrowths of plagioclase and quartz. Metamorphic plagioclase grains are also replaced by epidote. We interpret these microstructures to re¯ect strain-enhanced dissolution. The cores of many grains show asymmetric overgrowths with at least two generations of beards, all oriented on the ends of grains that face the extension direction. We interpret these textures to re¯ect precipitation of components dissolved by deformation-enhanced dissolution. While biotite and quartz probably deformed by dislocation creep, the overall deformation was accommodated by dissolution perpendicular to the shortening direction, and precipitation parallel to it. These chemical processes must have been activated at lower stresses than the dislocation creep predicted from extrapolations of data from experiments in dry rocks. Thus wet crust is likely to be weaker than calculated from these experimental studies. q 2002 Elsevier Science Ltd. All rights reserved. Keywords: Dissolution; Precipitation; Replacement creep; Pressure solution; Metamorphic reaction mechanism 1. Introduction shortening direction, as has been recognized in sandstones 7e.g. Blatt, 1992), metaconglomerates 7Mosher, 1976), and Evidence from naturally deformed rocks for the operation augen gneisses 7Simpson and Wintsch, 1989), commonly by of solution transfer is now well documented in sedimentary the concentration of less soluble minerals, `insoluble and low-grade metamorphic rocks 7e.g. Passchier and residue'. The dissolved components may precipitate as Trouw, 1996). Most signi®cantly, the development of quartz, feldspar, and even monazite, forming beards on cleavage and metamorphic fabric is now identi®ed to be a host grains 7Wintsch and Knipe, 1983; Williams et al., chemical process, albeit mechanically induced. The 1999). differentiation is thought to be caused by the dissolution In rocks under suf®ciently high-grade metamorphic of quartz and feldspar from developing mica-rich domains, conditions, dislocation glide and climbare activated and precipitation into growing quartz±feldspar domains. progressively in phyllosilicates, then in quartz, and ®nally Bulk chemical analytical studies do not support the proposi- in feldspars and pyroxenes 7Passchier and Trouw, 1996). tion that silica is removed from the developing slate at the Thus in dry rocks dislocation creep may be the most kilometer scale 7e.g. Wright and Platt, 1982). On the important deformation mechanism in the ductile deforma- contrary, micas and feldspars as well as quartz appear to tion of higher temperature 7.3008C), lower crustal rocks dissolve and reprecipitate locally 7centimeter scale), with 7Fig. 1). Where ¯uids are present, however, a ®eld of dis- only minor and trace elements that are differentially mobile solution and precipitation displaces the dislocation creep moving .100 m 7e.g. Wintsch et al., 1991). The sites of ®eld. This is best documented in low-grade rocks of dissolution are the surfaces normal to the principal ,200±3008C and is typically called `pressure solution' 7Fig. 1; Snoke et al., 1998). * Corresponding author. Tel.: 11-812-855-4018; fax: 11-812-855-7899. In this contribution we describe an orthogneiss meta- E-mail address: [email protected] 7R.P. Wintsch). morphosed to wet epidote±amphibolite facies conditions. 0191-8141/02/$ - see front matter q 2002 Elsevier Science Ltd. All rights reserved. PII: S0191-8141701)00100-6 1180 R.P. Wintsch, K. Yi / Journal of Structural Geology 24 /2002) 1179±1193 mechanism as suggested by the extrapolation of experimen- tal results from dry rocks 7see Fig. 1). 2. Geologic setting The samples analysed in this study were collected in the Glastonbury gneiss in north-central Connecticut 7Fig. 2). The Glastonbury gneiss is a complex of Ordovician volcanic and plutonic rocks that comprise the Bronson Hill terrane of central New England. It includes metamorphosed granitic, granodioritic, trondjemitic, and dioritic orthogneisses and schists. U±Pbgeochronology on zircon and sphene in this gneiss 7Leo et al., 1984; Aleinikoff et al., 2002) and on the Bronson Hill rocks in general 7Zartman and Leo, 1985; Tucker and Robinson, 1990) con®rm a Late Ordovician Fig. 1. Diagram showing the strength envelope of rocks in which frictional igneous crystallization age for these rocks. sliding ®rst in quartz-rich rocks, followed by feldspar-rich and ®nally by diopside-rich rocks gives way to plastic ¯ow at higher temperatures Apparently all Bronson Hill rocks were metamorphosed 7compiled from Kohlstedt et al. 71995) and Rybacki and Dresen 72000)). twice. In New Hampshire and Massachusetts, U±Pb The addition of a `pressure solution creep' ®eld is from Snoke et al. 71998). geochronology and 40Ar/39Ar hornblende thermochronology con®rms amphibolite facies metamorphism and cooling The foliation development, metamorphic differentiation, from the early Devonian Acadian orogeny. However, and grain shape in these rocks are all most easily explained Carboniferous to Permian 40Ar/39Ar mica and feldspar in terms of dissolution, precipitation, and replacement, with cooling ages document an Alleghanian greenschist facies relatively little evidence for dislocation creep. If these ¯uid- metamorphic overprint 7Harrison et al., 1989). In dependant deformation mechanisms are representative of Connecticut, the intensity of Alleghanian overprinting is processes operating in wet rocks at high grades, then dis- suf®ciently high that U±Pbages of all metamorphic sphene location creep may not be the prevailing deformation and all 40Ar/39Ar hornblende and mica cooling ages yield Fig. 2. Map of New England showing the distribution of lithotectonic terranes or zones, and the location of our study area within the Glastonbury gneiss of the Bronson Hill terrane of northern Connecticut. R.P. Wintsch, K. Yi / Journal of Structural Geology 24 /2002) 1179±1193 1181 Fig. 3. Plane light and crossed polarized light images of augen gneiss from the Glastonbury Complex. The images show 1 cm diameter grains of orthoclase 7Or) with Carlsbad twinning 7Cs Tw in B), locally embayed by myrmekite 7Myr). Darker layers in A, de®ned by biotite and epidote layers locally intergrown with amphibole 7Amp), outline an S±C fabric. These folia are separated by layers of clear quartz 7Qtz) and dusty plagioclase 7Pl). The locations of Figs. 4 and 5D are indicated. only Pennsylvanian to Permian ages 7Wintsch et al., 1993, these minerals are Ordovician igneous minerals, and some 1998; Coleman et al., 1997; Aleinikoff et al., 2002). are metamorphic minerals, produced by metamorphic reactions. Some participate directly in de®ning the foliation, and some do not. Consequently, each mineral is described 3. Observations separately below. The gneisses examined for this study are granodioritic, 3.1. Plagioclase containing centimeter±diameter K-feldspar crystals in a medium- to coarse-grained matrix of plagioclase, quartz, Plagioclase, the most abundant mineral in the gneiss, biotite, epidote, hornblende, K-feldspar, and sphene 7Fig. typically occurs as polygonal untwinned grains 100± 3). Biotite de®nes an inconspicuous foliation, and a very 300 mm in length 7Fig. 4), but some grains may be twice conspicuous NNW-plunging lineation parallel to prolate as large 7Fig. 5A). It tends to occur in layers and domains ma®c enclaves, such that the rocks are locally L-tectonites. from 1 to 5 mm wide and 10±15 mm long where it consti- S±C fabric relationships show that top moved to the SSE tutes .80% of the minerals present 7Figs. 3 and 4). Some 7Wintsch et al., 1998). Biotite is the most prominent mineral grains are equant 7Fig. 5B and C), but most are elongated de®ning the lineation, but elongate grains of plagioclase, parallel to the mineral lineation 7Fig. 5A and D). Aspect epidote and quartz also de®ne the lineation in their aspect ratios of 2:1 are typical, but they may be up to 4:1. These ratios and overgrowth patterns 7Figs. 3 and 4). Some of grains are universally zoned, with compositions ¯uctuating 1182 R.P. Wintsch, K. Yi / Journal of Structural Geology 24 /2002) 1179±1193 Fig. 4. A colorized backscattered electron image across the foliation shown in Fig. 3. The images were constructed by assembling and separately colorizing four images of the same regions, each adjusted to maximize compositional contrasts in plagioclase 7blue), orthoclase 7yellow), biotite, epidote, amphibole 7green) and sphene
Details
-
File Typepdf
-
Upload Time-
-
Content LanguagesEnglish
-
Upload UserAnonymous/Not logged-in
-
File Pages15 Page
-
File Size-