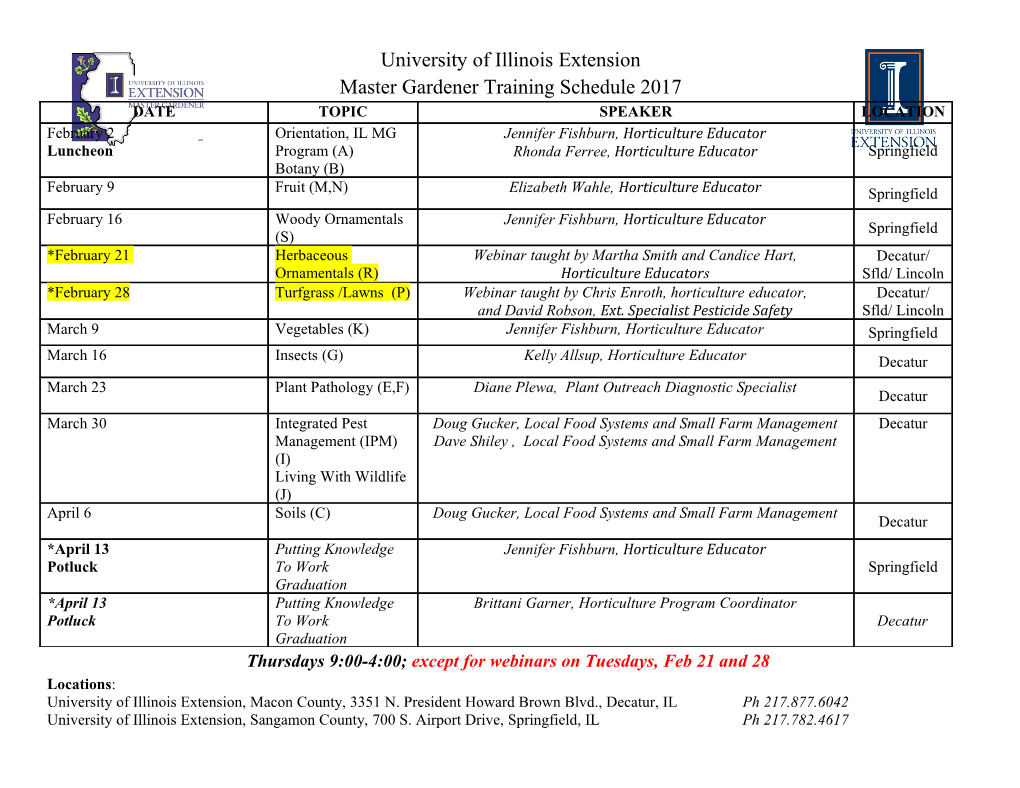
Undulatory Locomotion in Freshwater Stingray Potamotrygon Orbignyi: Kinematics, Pectoral Fin Morphology, and Ground Effects on Rajiform Swimming The Harvard community has made this article openly available. Please share how this access benefits you. Your story matters Citation Blevins, Erin Leigh. 2012. Undulatory Locomotion in Freshwater Stingray Potamotrygon Orbignyi: Kinematics, Pectoral Fin Morphology, and Ground Effects on Rajiform Swimming. Doctoral dissertation, Harvard University. Citable link http://nrs.harvard.edu/urn-3:HUL.InstRepos:9849992 Terms of Use This article was downloaded from Harvard University’s DASH repository, and is made available under the terms and conditions applicable to Other Posted Material, as set forth at http:// nrs.harvard.edu/urn-3:HUL.InstRepos:dash.current.terms-of- use#LAA ©2012 Erin Blevins All rights reserved. Professor George V. Lauder Erin Leigh Blevins Undulatory locomotion in freshwater stingray Potamotrygon orbignyi: kinematics, pectoral fin morphology, and ground effects on rajiform swimming Abstract Fishes are the most speciose group of living vertebrates, making up more than half of extant vertebrate diversity. They have evolved a wide array of swimming modes and body forms, including the batoid elasmobranchs, the dorsoventrally flattened skates and rays, which swim via oscillations or undulations of a broad pectoral fin disc. In this work I offer insights into locomotion by an undulatory batoid, freshwater stingray Potamotrygon orbignyi (Castelnau, 1855), combining studies of live animals, physical models, and preserved specimens. In Chapter 1, I quantify the three-dimensional kinematics of the P. orbignyi pectoral fin during undulatory locomotion, analyzing high-speed video to reconstruct three-dimensional pectoral fin motions. A relatively small portion (~25%) of the pectoral fin undulates with significant amplitude during swimming. To swim faster, stingrays increase the frequency, not the amplitude of propulsive motions, similar to the majority of studied fish species. Intermittently during swimming, a sharp, concave-down lateral curvature occurred at the fin margin; as the fin was cupped against the pressure of fluid flow this curvature is likely to be actively controlled. Chapter 2 employs a simple physical model of an undulating fin to examine the ground effects that stingrays may experience when swimming near a substrate. Previous research considering static air- and hydrofoils indicated that near-substrate locomotion offers a benefit to propulsion. Depending on small variations in swimming kinematics, undulating fins can swim iii faster near a solid boundary, but can also experience significant increases (~25%) in cost-of- transport. In Chapter 3, I determine how pectoral and pelvic fin locomotion are combined in P. orbignyi during augmented punting, a hybrid of pectoral and pelvic fin locomotion sometimes employed as stingrays move across a substrate. The timing of pectoral and pelvic fin motions is linked, indicating coordination of thrust production. Chapter 4 discusses pectoral fin structure and morphological variations within the fin, correlating morphology with the swimming kinematics observed in Chapter 1. Passive and active mechanisms may stiffen the anterior fin to create the stable leading edge seen during swimming; stingrays have converged on several structural features (fin ray segmentation and branching) shared by actinopterygian fishes. iv Table of Contents Introduction………………………………………………………………………………………..1 Chapter 1: Rajiform locomotion: three-dimensional kinematics of the pectoral fin surface during swimming by freshwater stingray Potamotrygon orbignyi…………………………………….....5 Chapter 2: Synchronized swimming: coordination of pelvic and pectoral fins during augmented punting by freshwater stingray Potamotrygon orbignyi….……………………………………...47 Chapter 3: Swimming near the substrate: a simple robotic model of stingray locomotion……...72 Chapter 4: Pectoral fin morphology of freshwater stingray Potamotrygon orbignyi……….….105 Appendix: Supplemental data from Chapter 1………………………………………………….151 v For my teachers. vi Acknowledgements I am extraordinarily lucky to have been advised by Dr. George V. Lauder. Thanks to the advice of Dr. Alison Sweeney, I attended the 2005 meeting of the Society for Integrative and Comparative Biology, walked into a few presentations about fish swimming, and the rest was history. Drs. Andrew Biewener, Stacey Combes, Farish Jenkins, Jr., and Karel Liem (in body and spirit) have offered great advice on my research and career as members of my thesis committee, as have past and present members of the Lauder Lab. In particular, my experience as a graduate student and teacher would not have been the same without my labmate Jeanette Lim. I am grateful to my students for everything I learned while standing at the front of a classroom, and through work with the Derek Bok Center for Teaching and Learning; to poets Joanna Klink and Liza Flum; and to Katie Humphry and the incomparable Nora Lally-Graves, my friends. Because it doesn’t go without saying, I am deeply thankful for the love and support of my partner, Anton Dorokhin, and my parents, Shelley and David Blevins, who have been with me all the way. My interest in biology is grounded in respect for and curiosity about animal life, and so in addition to the people mentioned above I honor the contributions of the stingrays and other fish involved in this work. vii Introduction Fishes move through the water by using their bodies and fins to push on the fluid that surrounds them, accelerating flows backward and creating thrust opposite to their direction of travel. Swimming fishes are able to create such thrust due to the density and viscosity of water, as they push off of a medium roughly eight hundred times more dense than air, and over an order of magnitude more viscous—but the same properties that allow fish to generate thrust lead to the creation of drag forces that resist motion (Vogel, 2003). Fishes have evolved myriad ways to accomplish locomotion, generating thrust and overcoming drag to move through the water and accomplish other necessary behaviors such as prey detection, predator avoidance, and breeding. Fish body forms are as diverse as their swimming modes. More than half of extant vertebrate species are fish, and within this diversity fish species span orders of magnitude in size and body flexibility, from microscopic larvae to giant whale sharks, and from the nearly- boneless hagfish that ties itself into knots, to the stiff-bodied boxfish with its unbending carapaces (Helfman, 2009). Classical descriptions of swimming modes grouped fishes by the predominant fins used to power propulsion, and the degree to which body undulation was involved in swimming (Breder, 1926). Subsequent research has expanded and revised these descriptions for many species, as new techniques such as high-speed videography, particle image velocimetry, robotic and computer modeling have allowed researchers to examine in greater detail the kinematics with which fish move, and the hydrodynamics that result from their interactions with the surrounding fluid. As three-dimensional analyses have become possible, the importance of considering 3-D interactions between fish and fluid has become increasingly apparent (Tytell et al., 2008; Flammang et al., 2011). At the same time, robotic models offer the 1 opportunity to simplify biological systems into physical models, studying the effects of particular parameters (e.g. shapes, movement patterns) in isolation (Lauder et al, 2011). Among Breder’s original classifications of fish swimming was the eponymous rajiform locomotion, which included swimming by skates (Rajidae) and other batoid elasmobranchs that swim via undulations or oscillations of expanded pectoral fins (Breder, 1926). Undulation and oscillation were initially considered as discrete modes, termed rajiform (after the skates) and mobuliform (after Mobulidae, the manta and devil rays) respectively (Webb, 1994). More recently, they have been recognized as two poles of a continuum defined by the number of waves present on a batoid’s pectoral fin during swimming (wavelength/pectoral disc length; Rosenberger, 2001). Batoids also exhibit a range of pectoral fin shapes, from the triangular fins of mobulids and other oscillators, with a high ratio of fin span to chord, to the rounder shapes of undulators, with a low ratio of fin span to chord (Webb, 1994; Rosenberger, 2001). The combination of diversity in pectoral disc shape and swimming kinematics results in a large parameter space for batoid locomotion. Even within a single undulatory species, the broad, flexible pectoral surface undulates in three dimensions, with the potential for waveforms to vary along fin chord and span. The ability to vary pectoral fin locomotor patterns is important for batoids. In many fishes, separate fin and body surfaces control separate aspects of locomotion: some may control torques while others produce thrust, and others are involved in maneuvering. In sharks, the body provides lift while the caudal fin drives propulsion, and the pectoral fins add additional lift during ascents (Wilga and Lauder, 2002). In batoids, the body and pectoral fins are essentially fused into one surface (Compagno, 1999). All locomotor functions and some besides, from steady swimming to maneuvering and even prey capture (Wilga et al., 2012) must be achieved by modulating the 2 shape of the pectoral waveform. However, there is one notable exception –despite
Details
-
File Typepdf
-
Upload Time-
-
Content LanguagesEnglish
-
Upload UserAnonymous/Not logged-in
-
File Pages165 Page
-
File Size-