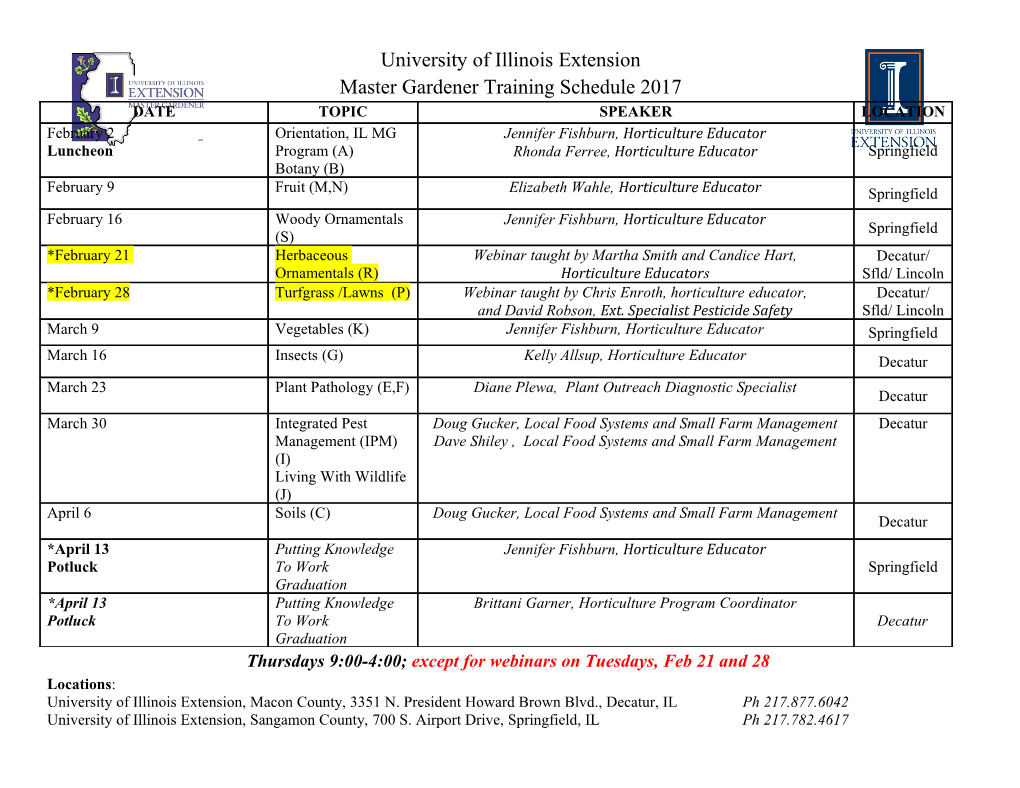
University of Amsterdam Institute for Theoretical Physics Master’s Thesis Hunting for Micro Black Holes Author: Supervisor: Lucas Ellerbroek Prof. dr. Erik Verlinde October 30, 2009 Alice laughed. “There’s no use trying,” she said: “one can’t believe impossible things.” “I daresay you haven’t had much practice,” said the Queen. “When I was your age, I always did it for half-an-hour a day. Why, sometimes I’ve believed as many as six impossible things before breakfast.” Lewis Carroll, Through the Looking Glass (And What Alice Found There), 1871 Cover illustration: Sir John Tenniel, “Alice’s Adventures in Wonderland”, 1865 Abstract In braneworld scenarios, the visible universe is pictured as a brane embedded in a higher- dimensional compact space, through which only the gravitational field propagates. In these scenarios, gravity could be the dominant force at TeV-scale energies. The discovery of hidden dimensions would therefore be a major breakthrough in finding a solution to the hierarchy problem. A phenomenological consequence of these scenarios is that black holes can exist at energy scales as low as 1 TeV. This leads to predictions about black hole events at future LHC experiments, that would prove the existence of hidden dimensions. This possibility sparked a lot of discussion among scientists, and a lawsuit was filed by worried citizens, afraid to be accreted by this black hole. However, careful study of Hawking radiation of TeV-scale black holes indicates that they evaporate very rapidly. Also, the signature of the radiation spectrum will probably be obscured by other emission effects. This narrows the chances of observing a black hole in a detector. In this research, a model is proposed of the creation of a black hole within a hot quark-gluon plasma. Under certain conditions, the plasma could be partly absorbed by the black hole, thus extending its lifetime. This could improve the chances of detecting a microscopic black hole. Contents 1 Introduction 5 2 Black holes in 4 dimensions 8 2.1 Formation ...................................... 8 2.1.1 Gravitational collapse ........................... 9 2.1.2 High-energy collisions ........................... 10 2.2 Properties ...................................... 13 2.2.1 The Unruh effect and Hawking radiation ................. 14 2.2.2 Superradiance ................................ 20 2.2.3 Schwinger discharge ............................ 22 2.3 Evaporation ..................................... 25 3 Braneworld scenarios 26 3.1 Motivation ..................................... 26 3.2 The ADD scenario ................................. 27 4Blackholesin4+n dimensions 32 4.1 Formation: high-energy collisions ......................... 32 4.2 Properties ...................................... 34 4.2.1 Schwarzschild radius ............................ 34 4.2.2 Hawking temperature ........................... 35 4.2.3 Greybody factors .............................. 36 4.3 Evaporation ..................................... 38 4.3.1 Balding phase ................................ 39 4.3.2 Spindown phase .............................. 40 4.3.3 Schwarzschild phase ............................ 40 4.3.4 Planck phase ................................ 44 4.3.5 Lifetime ................................... 44 5 Primordial black holes 46 5.1 Mass limits for PBHs in braneworld scenarios .................. 46 5.2 Primordial high-energy collisions ......................... 47 2 6 Black holes in the lab 51 6.1 The quark-gluon plasma .............................. 51 6.1.1 Creation ................................... 52 6.1.2 Cooling and Expansion .......................... 53 6.1.3 Hadronization ................................ 54 6.2 The QGP Absorption Model ........................... 54 6.2.1 Growth and decay ............................. 55 6.2.2 Numerical results .............................. 58 6.2.3 Theoretical Issues ............................. 64 6.2.4 The Gunfire Model ............................. 66 6.2.5 Feasibility .................................. 66 7 Conclusions 69 A Lorentz Force Lagrangian 72 B Emission coefficient for black holes with fixed mass 73 C Graphs 75 4 CONTENTS Notation The equations in this thesis are written in SI units unless specified otherwise. The reason for this is that the numerical results become more insightful. The following relation between the gravitational constant and the Planck mass is used: c G = 2 (0.1) Mpl The four-dimensional value of the Planck scale is denoted by Mpl.Foritshigher- dimensional equivalent the symbol M∗ is used. Chapter 1 Introduction With much anticipation, the scientific world watches the Large Hadron Collider in Geneva, where for the first time TeV-energies will be reached in particle experiments. Some theorists speculated (for example [1, 2, 3]) that one of the products created in these collisions might be a black hole. When these predictions were published, a big hype was spawn among scientists and non-scientists alike. The small black hole originally proposed became blown up to gargantuan proportions by the media. Headlines featured doomsday scenarios, and even a lawsuit was filed to pull financial support out of the project. Fortunately for the European Organization for Nuclear Research (CERN), the judge ruled that the Apocalypse lay ‘outside of her jurisdiction’. In the meanwhile, scientific evidence had been comprised by some of the leading theorists on this topic that there was nothing to worry about [4, 5]. The official safety assessment from CERN was endorsed by numerous leading physicists, including Stephen Hawking: The world will not come to an end when the LHC turns on. The LHC is absolutely safe. ... Collisions releasing greater energy occur millions of times a day in the earth’s atmosphere and nothing terrible happens [6]. Some questions that immediately come to mind are: how can black holes be fabricated at LHC? What will happen to them upon creation? Can they even be detected? These questions are the backbone of this thesis. To answer the first question: black holes are gravitational objects, so they can only exist at TeV-scales if gravity acted on this scale. And according to our current knowledge of nature, it does not. The relative weakness of gravity as opposed to the other three forces is called the hierarchy problem. It is one of the major outstanding problems in physics. Among its proposed solutions are theories called braneworld scenarios. In these scenarios, the scale of gravity, which is the Planck scale, is lowered down. This means that gravity becomes stronger on short distances than we would expect it to be ac- cording to Newtonian physics. This is explained by the existence of extra, hidden dimensions where only gravity propagates. Because some of the gravitational flux ‘leaks out’ to the extra dimensions, only a weak gravity force remains in the observable universe. In this way, the 5 6 CHAPTER 1. INTRODUCTION observed Planck scale is merely an effective value of a fundamental scale, which can be as low as the electroweak scale, around 1 TeV. In this line of reasoning, it comes as no surprise that should these scenarios represent reality, and gravity is so strong on such short distances, the creation of black holes may well occur at LHC experiments. Current measurements of gravity do not rule out these braneworld scenarios. Hence the excitement upon this prediction. The detection of a black hole would tell us much about the structure of spacetime, it would prove the existence of extra dimensions, and it might solve the hierarchy problem. Also, for the first time it would be possible to probe the quantum gravity regime. On the other hand, it might mean the end of particle physics as we know it, since if scattering events hide behind event horizons, none of them will be detected. The consequences for physics may be dire, but the Earth will not be obliterated by these microscopic black holes. The argument mentioned by Hawking, that cosmic ray observations tell us that black holes formed in collisions do not pose a threat to the Earth, is central in the safety assessment released by CERN. The expectation is that these black holes are so tiny, that they will evaporate through thermal radiation, originally proposed by and named after Hawking himself. Since the temperature of black holes is inversely proportional to their size, microscopic black holes will be extremely hot. They will decay rapidly in detectors, before they get the chance to accrete matter. It is even a question if we would even measure this radiation, since the spectrum is not exactly thermal. For this reason, it would be interesting to know if black holes produced at accelerators can somehow be made larger, before they get a chance to evaporate. One possibility might be to force the black hole to absorb large quantities of a hot plasma. It is possible that this has already happened in the early radiation-dominated universe. In the future we might even be able to simulate this situation in a laboratory environment. In particular, we could make a black hole inside a very hot quark-gluon plasma, making the hole grow by absorption. This thesis explores the exotic events that TeV-scale Gravity (TeVG) implied by braneworld scenarios makes possible. It follows the storyline laid out in the paragraphs above. In chapter 2, the general properties of black holes in four dimensions are reviewed. The different means of particle emission by black holes, including Hawking radiation, are derived. The differences between large and small black holes are made clear. It is also
Details
-
File Typepdf
-
Upload Time-
-
Content LanguagesEnglish
-
Upload UserAnonymous/Not logged-in
-
File Pages82 Page
-
File Size-