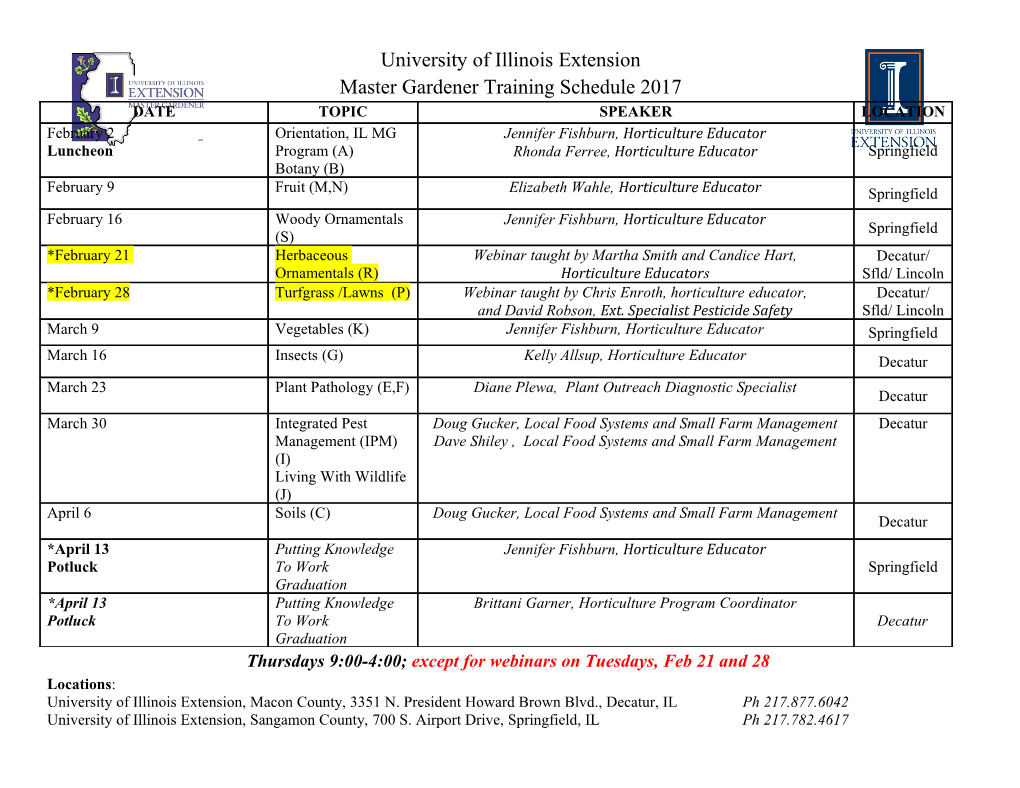
Theoretical calculations on the structural, electronic and optical properties of bulk silver nitrides Mohammed S. H. Suleiman1,2, ∗ and Daniel P. Joubert1, † 1School of Physics, University of the Witwatersrand, Johannesburg, South Africa. 2Department of Physics, Sudan University of Science and Technology, Khartoum, Sudan. (Dated: January 1, 2013) We present a first-principles investigation of structural, electronic and optical properties of bulk crystalline Ag3N, AgN and AgN2 based on density functional theory (DFT) and many-body pertur- bation theory. The equation of state (EOS), energy-optimized geometries, cohesive and formation energies, and bulk modulus and its pressure derivative of these three stoichiometries in a set of twenty different structures have been studied. Band diagrams and total and orbital-resolved density of states (DOS) of the most stable phases have been carefully examined. Within the random-phase approximation (RPA) to the dielectric tensor, the single-particle spectra of the quasi electrons and quasi holes were obtained via the GW approximation to the self-energy operator, and optical spec- tra were calculated. The results obtained were compared with experiment and with previously performed calculations. CONTENTS published works5. Due to its early discovery, copper ni- tride may now be considered as the most investigated 6 I. Introduction 1 among the late TMNs . On the other hand, the nitride of silver, the next el- II. Calculation Methods 2 ement to copper in group 11 of the periodic table, has 7,8 A. Stoichiometries and Crystal Structures 2 been known for more than two centuries . However, de- B. Electronic Relaxation Details 2 spite its earlier discovery, silver nitride may be the least theoretically studied solid in the late TMNs family. Ex- C. Geometry Relaxation and EOS 3 8,9 8 D. Formation Energy 3 perimental efforts to investigate structural , electronic and formation7,9–11 properties of silver nitrides have been E. GWA Calculations and Optical Properties 3 made by some researchers. 9 8 III. Results and Discussion 4 In 1949, Hahn and Gilbert carried out the first struc- A. EOS and Relative Stabilities 4 tural study on the reported stoichiometry, Ag3N. They claimed an fcc structure with a = 4.369 A˚ and Z = 4/3 B. Volume per Atom and Lattice Parameters 7 (i.e. 4 Ag atoms in the unit cell). A long time later in C. Bulk Modulus and its Pressure Derivative 8 1982, Haisa12 suggested that the Ag atoms are located D. Formation Energies 8 at the corners and face centers of the unit cell, while the E. Electronic Properties 9 N atoms, which may be statistically distributed in the F. Optical Properties 12 octahedral interstices, were given no definite positions12. According to the calculated N radius, Ag3N can be IV. Conclusions 12 described as an ionic compound, and recent ab initio calculations on the proposed structure revealed insulat- Acknowledgments 12 ing characteristics with a fundamental band gap close to 1.35 eV . On the other hand, due to the similar lattice of References 13 the parent Ag and the easily separated N as N2, it can also be argued that this compound is a metal, supporting its black color8. I. INTRODUCTION Under ordinary conditions7, it was found that sil- arXiv:1212.6507v1 [cond-mat.mtrl-sci] 28 Dec 2012 13 ver can form Ag3N from ammoniacal solutions of sil- It is well-known now that late transition-metal nitrides ver oxide7,8. The black metallic-looking solid outcome, 7,14 (TMNs) usually possess interesting properties leading Ag3N, is an extremely sensitive explosive compound . to a variety of potential technological applications1–3. It may explode due to the slightest touch, even from the Hence, a significant number of quantum mechanical ab impact of a falling water droplet14, but it is relatively initio calculations of the structural and physical prop- easy to handle under water or ethanol8. The explosive erties of this family of materials have appeared in the power is due to the energy released during the decompo- literature. sition reaction: 4 Since Juza and Hahn succeeded to synthesize Cu3N in 2Ag N 6Ag + N2 . (1) 1939, copper nitrides have been produced through vari- 3 −→ ous techniques and their properties and applications have Even in storage at room temperature, this solid com- been the subject of many theoretical and experimental pound decomposes slowly according to Eq. 1 above8,14. 2 From a thermochemical point of view, it was found that the face-centered cubic structure of NaCl (B1), the sim- there is no stable intermediate stage in this decomposi- ple cubic structure of CsCl (B2), the face-centered cubic tion, but there may be a metastable intermediate species structure of ZnS zincblende (B3), the hexagonal structure 7 (phase) with a remarkably low decomposition rate . At of NiAs (B81), the hexagonal structure of BN (Bk), the this point, it may be worth mentioning that the ther- hexagonal structure of WC (Bh), the hexagonal structure mochemistry of silver nitride systems is not fully docu- of ZnS wurtzite (B4), the simple tetragonal structure of mented in standard handbook data7. PtS cooperite (B17), and the face-centered orthorhombic In their 1991 work, Shanley and Ennis7 stated: “Many structure of TlF (B24). of the samples ... did not survive the minimum han- AgN2 was studied in the following nine structures: the dling required to move them, container and all, to the face-centered cubic structure of CaF2 fluorite (C1), the X-ray stage. ... More vigorously explosive samples prop- simple cubic structure of FeS2 pyrite (C2), the simple agated throughout their mass leaving no visible residue. orthorhombic structure of FeS2 marcasite (C18) and the Even among supersensitive materials, silver nitride is a simple monoclinc structure of CoSb2 (CoSb2). striking example of a compound “teetering on the edge of existence”. Under the circumstances, we did not suc- ceed in developing data on the proportion of silver nitride required for explosive behavior in these mixtures.” Thus, beside the potential hazard to lab workers due to B. Electronic Relaxation Details its sensitive explosive behavior, characterization of silver nitride is hindered by its extremely unstable (endother- 7,8 In this work, electronic structure spin density func- mic) nature , and we are presented with an incomplete tional theory (SDFT)19,20 calculations as implemented picture of structural, electronic and optical properties of in the VASP code21–26 have been employed. To self- this material. Surprisingly, this lack of detailed knowl- consistently solve the Kohn-Sham (KS) equations27 edge of many physical properties of silver nitride stimu- lated only very few published ab initio studies. In the present work, first-principles calculations were ~2 n(r′) 2 + dr′ + V (r) carried out to investigate the lattice parameters, equation r r′ ext ( − 2me ∇ of state, relative stabilities, phase transitions, electronic Z | − | (2) and optical properties of silver nitrides in three different σ,k r σ,k r σ,k σ,k r chemical formulae and in various crystal structures. Cal- +VXC [n( )] ψi ( )= ǫi ψi ( ), culation methods are described in Sec. II. In Sec. III, ) results are presented, discussed and compared with ex- periment and with previous calculations. The article is where i, k and σ are the band, k-point and spin in- concluded with some remarks in Sec. IV. dices, receptively, VASP expands the pseudo part of the σ,k r KS one-particle spin orbitals ψi ( ) on a basis set of plane-waves (PWs). Only those PWs with cut-off en- II. CALCULATION METHODS ergy Ecut 600 eV have been included. The Brillouin zones were≤ sampled using Γ-centered Monkhorst-Pack28 A. Stoichiometries and Crystal Structures 17 17 17 meshes. Any increase in the Ecut value or in× the density× of the k-mesh produces a change in the To the best of our knowledge, the only experimen- total energy less than 3 meV/atom. For static calcula- tally reported stoichiometries of Ag-N compounds are tions, partial occupancies were set using the tetrahedron 7 7 29–31 Ag3N and AgN3 . However, previous ab initio stud- method with Bl¨ochl corrections , while the smearing 8 8,15 32 ies on Ag-N compounds considered Ag4N , Ag3N , method of Methfessel-Paxton (MP) was used in the 15 15,16 15,17 Ag2N , AgN and AgN2 in some cubic struc- ionic relaxation, and Fermi surface of the metallic phases tures only. Consideration of stoichiometries other than has been carefully treated. The Perdew-Burke-Ernzerhof the reported ones is probably due to the fact that many (PBE) parametrization33–35 of the generalized gradi- transition metals nitrides (TMs) are known to form more ent approximation (GGA)36–38 was employed for the 18 σ,k r than one nitride . Hence, our interest in investigating exchange-correlation potentials VXC [n( )]. The imple- 26,39 AgN and AgN2 is based on this fact. mented projector augmented wave (PAW) method For Ag3N, we consider the following seven structures: was used to describe the core-valence interactions Vext(r), 10 1 2 3 the face-centered cubic structure of AlFe3 (D03), the sim- where the 4d 5s electrons of Ag and the 2s 2p elec- ple cubic structure of Cr3Si (A15), the simple cubic struc- trons of N are treated explicitly as valence electrons. ture of the anti-ReO3 (D09), the simple cubic structure While for these valence electrons only scalar kinematic of Ag3Au (L12), the body-centered cubic structure of relativistic effects are incorporated, the PAW potential 25 CoAs3 (D02), the hexagonal structure of ǫ-Fe3N, and the treats the core electrons in a fully relativistic fashion . trigonal (rhombohedric) structure of RhF3. No spin-orbit interaction of the valence electrons has For AgN, the following four structures were considered: been considered.
Details
-
File Typepdf
-
Upload Time-
-
Content LanguagesEnglish
-
Upload UserAnonymous/Not logged-in
-
File Pages15 Page
-
File Size-