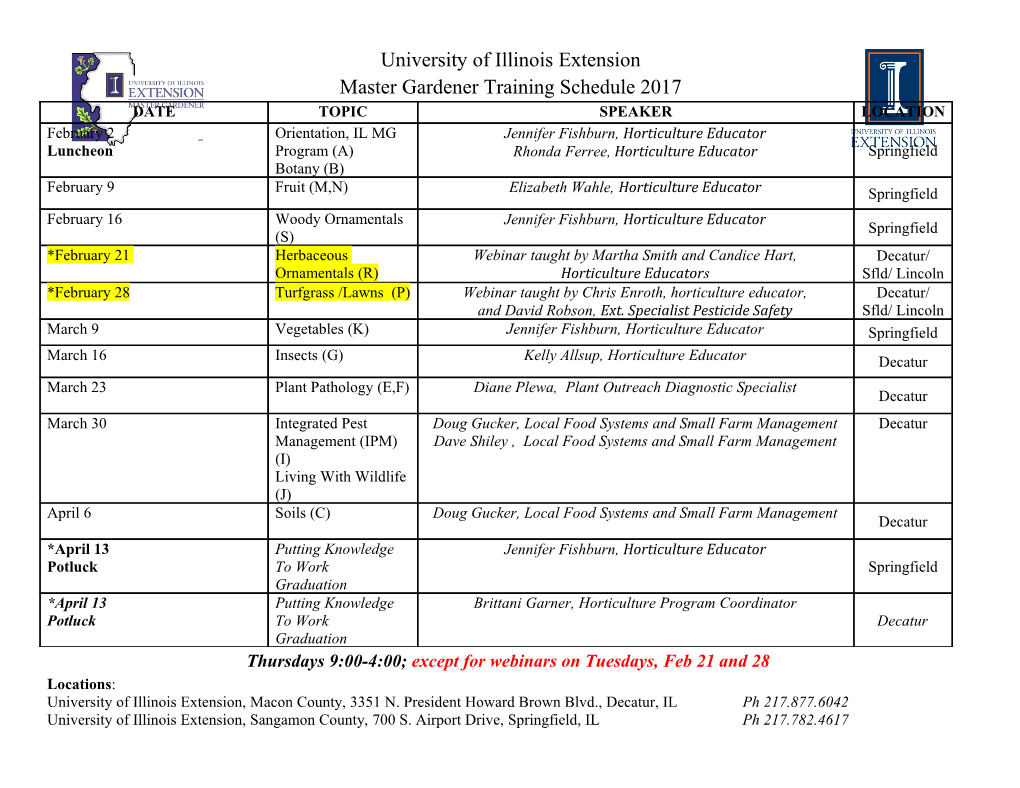
Examples of Semiconductor Lasers Quantum well lasers Advanced lasers: Quantum dot lasers Next time: Advanced lasers QCLs Single mode lasers DFB lasers VCSELs Reminder from last lecture: Design considerations for laser diode performance • Low threshold current – low threshold can be generated by electronic devices which can be modulated at high speed to provide a high speed modulation in the output (1) reducing the active layer thickness ↣ Quantum-Well (~ 50 - 100 Å), Strained Quantum-Well (2) cavity design • Lateral confinement reduce the lateral dimension of the Fabry-Perot cavity (1) Stripe geometry (Gain-guided cavity) (2) Buried heterostructures • Selective Optical Cavity – to reduce the laser linewidth (1) Distributed Feedback (DFB) structures (2) Vertical cavity surface emitting lasers (VCSELs) Evolution of the threshold current of the semiconductor lasers Quantum Well Laser • Constant 2D density of states means a large concentration of electrons can easily occur at E1 (and holes at the minimum valence band energy) • Population inversion occurs quickly without the need for a large current to bring a large number of electrons • Benefits: Threshold current reduced, linewidth is narrower Multiple Quantum Well (MQW) Laser • Several single quantum wells are coupled into a “multiple quantum well (MQW)” structure. • The significantly reduced temperature sensitivity of MQW lasers has been related to the staircase density of states distribution and the distributed electron and photon distributions of the active region. • The optical confinement helps to contain the otherwise large losses from a narrow active region, leading to low threshold currents. Bandgap engineering: Visible-UV-IR range Red QW Laser Diode Diagram of red GaInP DQW laser Diagram showing the alloy composition through the layer structure of a two-well, separate confinement (AlyGa1−y)In1−xP quantum well laser. The vertical distance axis is not to scale: the wells are each about 6.5 nm wide, the y=0.5 waveguide core is about 200 nm thick, and the cladding layers are each about 1 μm thick. Violet QW Laser Diode Diagram of deep violet InGaN DQW laser structures From: “Performance enhancement of deep violet indium gallium nitride double quantum well lasers using delta barrier close to electron blocking layer”, J. Nanophoton. 2012;6(1):063514-1-063514-12. doi:10.1117/1.JNP.6.063514. Modes: longitudinal and transverse Longitudinal modes Transverse modes Laser waveguides design for transverse confinement • Vertical confinement • Lateral confinement – Gain-guided – Index guided: ridges, ribs – Buried heterostructure lasers Vertical confinement Graded Index Separate Confinement Heterostructure (GRINSCH) Laser • GRaded INdex Separate Confinement Heterostructure (GRINSCH) Laser • A narrower carrier confinement region (d) of high recombination is separated from a wider optical waveguide region • Optical confinement can be optimized without affecting the carrier confinement • GRINSCH-SQW and GRINSCH-MQW • The threshold current for a GRINSCH is much lower than that of a DH laser GRINSCH Laser Lateral confinement Efficient operation of a laser diode requires reducing the # of lateral modes, stabilizing the gain for lateral modes as well as lowering the threshold current. These are met by structures that confine the optical wave, carrier concentration and current flow in the lateral direction. Important types of laser diodes are: gain-guided, positive index guided, and negative index guided. Gain guided: optical gain is highest where current density is greatest • Stripe contact increases current density in the active region. Cleaved reflecting surface • The widths of the active region or W the optical gain region is defined by current density from the stripe L Stripe electrode Oxide insulator p-GaAs (Contacting layer) p-Al Ga As (Confining layer) x 1-x p-GaAs (Active layer) n-Al Ga As (Confining layer) 2 x 1-x 1 3 Substrate n-GaAs (Substrate) Current pathsSubstrate Electrode Elliptical Cleaved reflecting surface laser Active region where J > J . beam th (Emission region) Schematic illustration of the the structure of a double heterojunction stripe contact laser diode © 1999 S.O. Kasap, Optoelectronics (Prentice Hall) Ridge laser Index guided: optical power confined to waveguide Electrode Oxide insulation p + -AlGaAs (Contacting layer) p -AlGaAs (Confining layer) n- AlGaAs p -GaAs (Active layer) n -AlGaAs (Confining layer) n -GaAs (Substrate) Schematic illustration of the cross sectional structure of a buried heterostructure laser diode. © 1999 S.O. Kasap, Optoelectronics (Prentice Hall) • Active layer is surrounded by lower index AlGaAs and behaves like a dielectric waveguide • Ensures that photons are confined to the active or optical gain region • Increases rate of stimulated emission Buried heterostructure laser Laser Diodes (temperature characteristics) The output characteristics of an LD are sensitive to temperature. =>As temperature increases threshold current increases exponentially. Output spectrum also changes. A single mode LD will mode hop (jump to a different mode) at certain temperatures. This results in a change of laser oscillation wavelength. increases slowly due to small change in refractive index and cavity length. Po (mW) 10 0 C 50 C 8 25 C 6 4 2 0 I (mA) 0 20 40 60 80 Output optical power vs. diode current as three different temperatures. The threshold current shifts to higher temperatures. © 1999 S.O. Kasap, Optoelectronics (Prentice Hall) Single mode Single mode Multimode 788 (a) (b) (c) 786 784 o 782 (nm) 780 Mode hopping 778 776 20 30 40 50 20 30 40 50 20 30 40 50 Case temperature ( C) Case temperature ( C) Case temperature ( C) Peak wavelength vs. case temperature characteristics. (a) Mode hops in the output spectrum of a single mode LD. (b) Restricted mode hops and none over the temperature range of interest (20 - 40 C). (c) Output spectrum from a multimode LD. © 1999 S.O. Kasap, Optoelectronics (Prentice Hall) Laser Diodes (temperature characteristics) Remedies if mode hopping is undesirable: 1. Adjust device structure. 2. Implement thermoelectric (TE) cooler. Gain guided LDs inherently have many modes therefore the wavelength vs. temperature behaviour tends to follow the bandgap (optical gain curve as opposed to the cavity properties). Advanced semiconductor lasers Quantum dot (QD) lasers Evolution of the threshold current of the semiconductor lasers 0-D (Quantum dot): An artificial atom Areal density: E (E) (E Ei ) Ei Theoretical quantum dots (a) Structure of a 4nm-high, 10 nm-wide hexagonal GaN quantum dot embedded in AlN. (b) Profile of the conduction band edge. (c) Maps of the dot electron ground state, (d) Map of the first excited state. In Stranki-Krastanov growth of QDs: strain-mediated intra- and inter-layer interactions between the QDs Aligned array of GaN QDs in AlN QDL – Predicted Advantages • Wavelength of light determined by the energy levels not by bandgap energy: – improved performance & increased flexibility to adjust the wavelength • Maximum material gain and differential gain • Small volume: – low power high frequency operation – large modulation bandwidth • Superior temperature stability of I threshold I threshold (T) = I threshold (T ref).exp ((T-(T ref))/ (T 0)) – High T 0 decoupling electron-phonon interaction by increasing the intersubband separation. – Undiminished room-temperature performance without external thermal stabilization • Suppressed diffusion of non-equilibrium carriers Reduced leakage QDL – Basic characteristics • An ideal QDL consists of a 3D-array of dots with equal size and shape • Surrounded by a higher band-gap material – confines the injected carriers. • Embedded in an optical waveguide – Consists lower and upper cladding layers (n-doped and p-doped shields) Edge emitting QDL http://qdlaser.com/ QDL – Application Requirements • Same energy level – Size, shape and alloy composition of QDs close to identical – Inhomogeneous broadening eliminated real concentration of energy states obtained • High density of interacting QDs – Macroscopic physical parameter light output • Reduction of non-radiative centers – Problem for nanostructures made by high-energy beam patterning since damage occurs during fabrication • Electrical control – Electric field applied can change physical properties of QDs – Carriers can be injected to create light emission Comparison of QD Laser with QW laser http://qdlaser.com/ QD Laser vs. QW Laser • Comparison of efficiency: QWL vs. QDL Bottlenecks • First, the lack of uniformity. Single dot • Quantum Dots density is insufficient • the lack of correlation between QDs FWHM = 20-30 meV Ensemble of QDs Breakthroughs Fujitsu Temperature Independent QD laser 2004 Fujitsu's quantum dot laser fires data at 25Gbps (2010) Temperature dependence of light-current characteristics Breakthroughs InP instead of GaAs Can operate on ground state for much shorter cavity length High T0 is achieved First buried DFB DWELL operating at 10Gb/s in 1.55um range Surprising narrow linewidth-brings a good phase noise and time- jitter when the laser is actively mode locked Alcatel Thales III–V Laboratory, France 2006 High-Performance Quantum Dot Lasers and Integrated Optoelectronics on Si Market demand of QD lasers Microwave/Millimeter wave transmission with optical fibers Datacom network Telecom network QD Lasers Optics Promising properties High speed Advantages quantum dot lasers Directly Modulated Quantum •Datacom application Dot Lasers •Rate of 10Gb/s Mode-Locked Quantum Dot •Short
Details
-
File Typepdf
-
Upload Time-
-
Content LanguagesEnglish
-
Upload UserAnonymous/Not logged-in
-
File Pages43 Page
-
File Size-