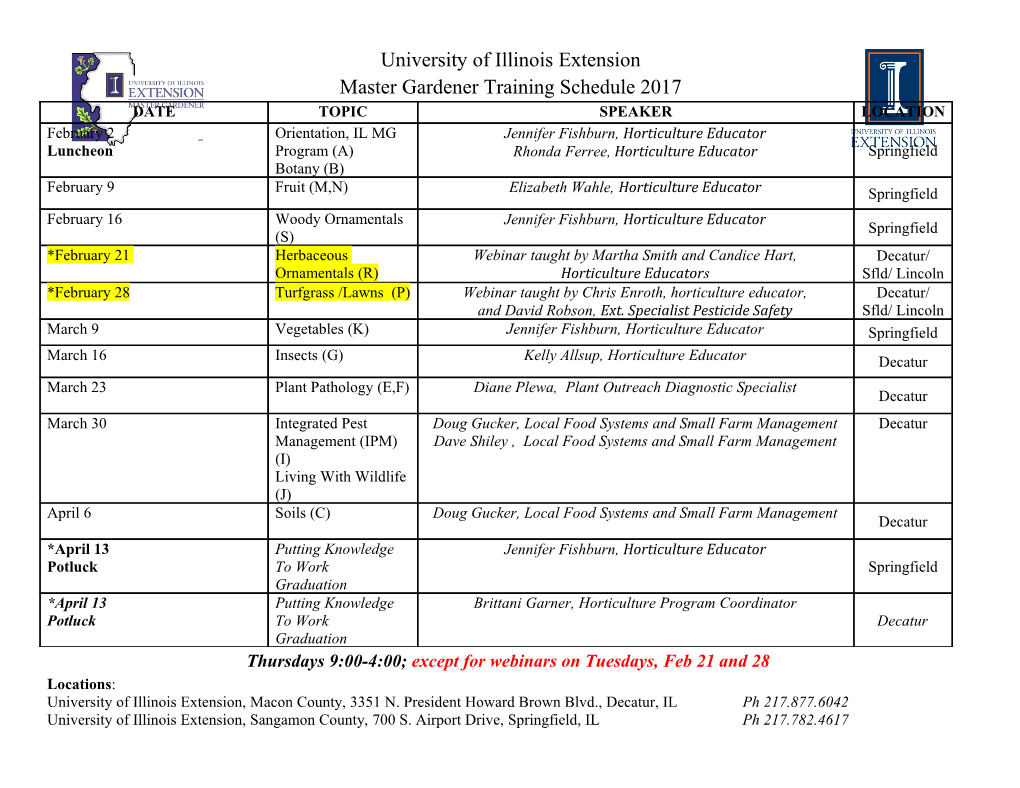
SEARCH FOR EXTRATERRESTRIAL LIFE USING CHIRAL MOLECULES: MANDELATE RACEMASE AS A TEST CASE A Thesis Presented to The Academic Faculty by Tracey Lyn Thaler In Partial Fulfillment of the Requirements for the Degree Doctor of Philosophy in the School of Chemistry & Biochemistry Georgia Institute of Technology May 2007 SEARCH FOR EXTRATERRESTRIAL LIFE USING CHIRAL MOLECULES: MANDELATE RACEMASE AS A TEST CASE Approved by: Dr. Andreas S. Bommarius, Advisor Dr. Christoph J. Fahrni School of Chemical & Biomolecular School of Chemistry & Engineering Biochemistry Georgia Institute of Technology Georgia Institute of Technology Dr. Rick P. Trebino Dr. Phillip R. Gibbs School of Physics Chief Science Officer Georgia Institute of Technology Stheno Corporation Dr. Donald F. Doyle School of Chemistry & Biochemistry Georgia Institute of Technology Date Approved: 03-28-07 ACKNOWLEDGEMENTS First and foremost I would like to thank my advisor Andy Bommarius for his support and encouragement over the past six and half years. I am also thankful to my thesis committee members: Donald Doyle, Christoph Fahrni, Phillip Gibbs and Rick Trebino for their patience as well as guidance. I would especially like to the thank Phillip Gibbs, who was not only the mastermind behind my thesis topic, but has always been encouraging in suggesting ideas to enhance my work. I would also like to thank my lab members past and present. Karen Polizzi has been a wonderful friend and colleague. We had many great conversations about α/β-barrel enzymes, which led to enhance my knowledge in this area. James Broering, also a great friend, has been very supportive of my efforts and helpful in teaching me about DSC, also I have found his cynical views refreshing. Javier Chaparro-Riggers was a great help in the development of the fourth Aim of this thesis as well as encouraging throughout the past two years. I would like to thank Janna Blum and Thomas Rogers for proofreading parts of my thesis. Also, thanks to Rongrong Jiang, Eduardo Vazquez and Bernard Loo, whom I have enjoyed working along side of in the lab. I have also had support from members of the Doyle lab. Especially Bahareh Azizi who has been encouraging in more ways than she will ever iii know, she is an awesome person and I hope she realizes the great potential of her future. Also, I had some great times with Lauren Schwimmer, and I am thankful to her for helping me when I had chemistry questions. Terry Watt has been an extremely useful source for discussing analysis of irreversible DSC data, he is also always willing to help when I have a question. Kenyetta Johnson and Priyanka Rohatgi have been great friends. I am very thankful to Christian Uehara who wrote the computer code for the DSC data analysis. I appreciate all of the hard work he spent on it. I am also thankful for the Astrobiology meetings with Rick Trebino and Neeraj Kothari, this project has increased my scientific knowledge in the field. I would also like to thank Brian Lynch from the School of Biology at Georgia Tech for always being able to provide me with adequate sequences. I would like to also thank Perry Mars for sequencing at the FAME sequencing center (Emory University). Further I would like to thank my family for their support, most of all my sister Lisa Thaler. She has been the most supportive person in my life throughout this entire endeavor and I am very lucky to have her in my life. I am most thankful for Lisa’s help with proofreading my thesis. I would also like to thank Donald Murphy for his technological support with my computer as well as his friendship. Margaret and Hadley Wellborn have been extremely supportive throughout the past two years and I am iv especially thankful for their help with Lily at all times. William Wellborn has been an astonishing addition to my life and I am grateful for all that he has done for me both in and out of the lab. He is a wonderful person and I am lucky to have him in my life. v TABLE OF CONTENTS Page ACKNOWLEDGEMENTS iii LIST OF TABLES xii LIST OF FIGURES xiv SUMMARY xviii CHAPTER 1: INTRODUCTION: DEVELOPING A BENCHMARK TO TEST A NOVEL POLARIMETER 1 1.1 REFERENCES 11 CHAPTER 2: BACKGROUND: THE SEARCH FOR EXTRATERRESTRIAL LIFE USING HOMOCHIRALITY AND RACEMIZATION 18 2.1 Origin of Life 18 2.2 Regions of Solar System to Explore 21 2.2.1 Mars 22 2.2.2 Europa 24 2.2.3 Enceladus 25 2.2.4 Titan 26 2.3 Origin of Homochirality 27 2.4 Racemization and the Search for Extraterrestrial Life 29 2.5 The Model System 30 2.6 MR Background 32 2.6.1 α/β Barrel Enzymes 32 vi 2.6.1.1 The Structure of α/β-Barrel Enzymes 32 2.6.1.2 Evolution of α/β Barrel Enzymes 34 2.6.2 Enolase Superfamily 37 2.7 Summary 39 2.8 REFERENCES 41 CHAPTER 3: CLONE, OVEREXPRESS, PURIFY, AND CHARACTERIZE MANDELATE RACEMASE (MR) FROM PSEUDOMONAS PUTIDA 50 3.1 Background 50 3.1.1 MR Protein Purification 50 3.1.2 MR Assays 51 3.1.3 Kinetics and Thermodynamics of MR 53 3.1.4 Other Substrates and Inhibitors 55 3.1.4.1 Studies Investigating Mandelate Analogs 55 3.1.5 Mechanism for MR 58 3.1.6 Metal Requirement for MR 61 3.1.7 Aim 1 62 3.2 Materials and Methods 63 3.2.1 Materials 63 3.2.2 MR Expression/Purification from P. putida 63 3.2.3 General DNA Techniques 64 3.2.4 Cloning, Fermentation, and Expression 65 3.2.5 Purification of Recombinant Protein 66 3.2.6 Apo-Enzyme 67 3.2.7 Enzyme Assay 67 3.2.8 Polarimetric Measurements 68 vii 3.3 Results 68 3.3.1 MR Cloning, Overexpression, and Purification 71 3.3.2 Apo-Enzyme Studies 73 3.3.3 Kinetic Studies 74 3.3.4 Temperature Studies 77 3.4 Discussion 81 3.5 Summary and Conclusion 83 3.6 REFERENCES 85 CHAPTER 4: STUDY OF THE IRREVERSIBLE THERMAL DENATUATION OF MANDELATE RACEMASE USING DIFFERENTIAL SCANNING CALORIMETRY 88 4.1 Introduction 88 4.2 Background 90 4.2.1 DSC to measure enzyme thermostability 90 4.2.2 Analysis of irreversible thermal denaturation 92 4.2.3 Models 94 4.2.3.1 Two-state irreversible model 94 4.2.3.2 Lumry-Eyring model 98 4.2.3.3 Consecutive two-step irreversible model 101 4.2.4 Thermostability of mandelate racemase 103 4.3 Materials and Methods 105 4.3.1 Materials 105 4.3.2 DSC measurements 105 4.3.3 CD measurements 105 viii 4.3.4 Analysis of data 105 4.4 Results and Discussion 107 4.4.1 Scan Rate Dependence 107 4.4.2 Protein concentration dependence 108 4.4.3 Investigation of enthalpy values 110 4.4.4 Application of the three kinetic models 111 4.5 Conclusion 119 4.6 REFERENCES 121 CHAPTER 5: INVESTIGATION OF MR REACTIVITY AT LOW TEMPERATURES IN NON-AQUEOUS MEDIA 127 5.1 Background 127 5.1.1 Enzymatic reactions at low temperatures 128 5.1.1.1 Temperature effects 130 5.1.2 Biocatalysts in non-aqueous media 131 5.1.2.1 Organic solvents 133 5.1.2.2 High concentration salt solutions 134 5.1.2.2.1 Protein deactivation 134 5.1.2.3 Water-in-oil microemulsions 135 5.1.3 MR and organic solvents 136 5.2 Materials and methods 136 5.2.1 Materials 136 5.2.2 Organic solvent systems 137 5.2.3 Concentrated salt solutions 138 5.2.3.1 Salt deactivation experiments 139 ix 5.2.4 Water-in-oil microemulsion systems 139 5.3 Results 140 5.3.1 MR in organic solvents 140 5.3.2 MR in concentrated ammonium salts 143 5.3.2.1 Deactivation of MR in ammonium salts 147 5.3.3 MR in water-in-oil microemulsions 150 5.4 Discussion 151 5.5 Conclusions 154 5.6 REFERENCES 157 CHAPTER 6: EVOLUTION OF ENZYMATIC ACTIVITY IN THE ENOLASE SUPERFAMILY: THREE MUTATIONS CREATE CROSS-REACTIVITY BETWEEN TWO SUBGROUPS 162 6.1 Introduction 162 6.2 Background 166 6.2.1 Evolution Models 166 6.2.2 Enolase superfamily 170 6.2.2.1 Divergent evolution in enolase superfamily 172 6.3 Materials and Methods 176 6.3.1 Materials 176 6.3.2 Bacterial Strains and Growth Conditions 176 6.3.3 General DNA Techniques 177 6.3.4 Overlap Extension Method 178 6.3.5 Synthesis of N-acetyl-phenylglycine 179 6.3.6 Superimposition of NAAR on MR 179 x 6.3.7 Polarimetric assay for MR activity 180 6.3.8 Polarimetric assay for racemase activity 180 6.4 Results and discussion 181 6.4.1 MR shows phenylglycine activity 181 6.4.2 Substrates to investigate 182 6.4.3 Mutations to increase the size of MR active site 183 6.4.3.1 Substrate specificity of variants 185 6.4.3.2 Effects of the E317 variants 190 6.4.3.3 Reduction of clash from N-succinyl phg 191 6.4.4 Flexibility of the active site residues 194 6.4.5 Evolution of the enolase superfamily 197 6.4.6 Divergent evolution models 199 6.5 Conclusion 201 6.6 REFERENCES 204 CHAPTER 7: CONCLUSIONS AND FUTURE WORK 209 7.1 Conclusions 209 7.2 Future Work 212 7.3 REFERENCES 215 xi LIST OF TABLES Page Table 2–1 Alpha/Beta Barrel Enzyme Families.
Details
-
File Typepdf
-
Upload Time-
-
Content LanguagesEnglish
-
Upload UserAnonymous/Not logged-in
-
File Pages234 Page
-
File Size-