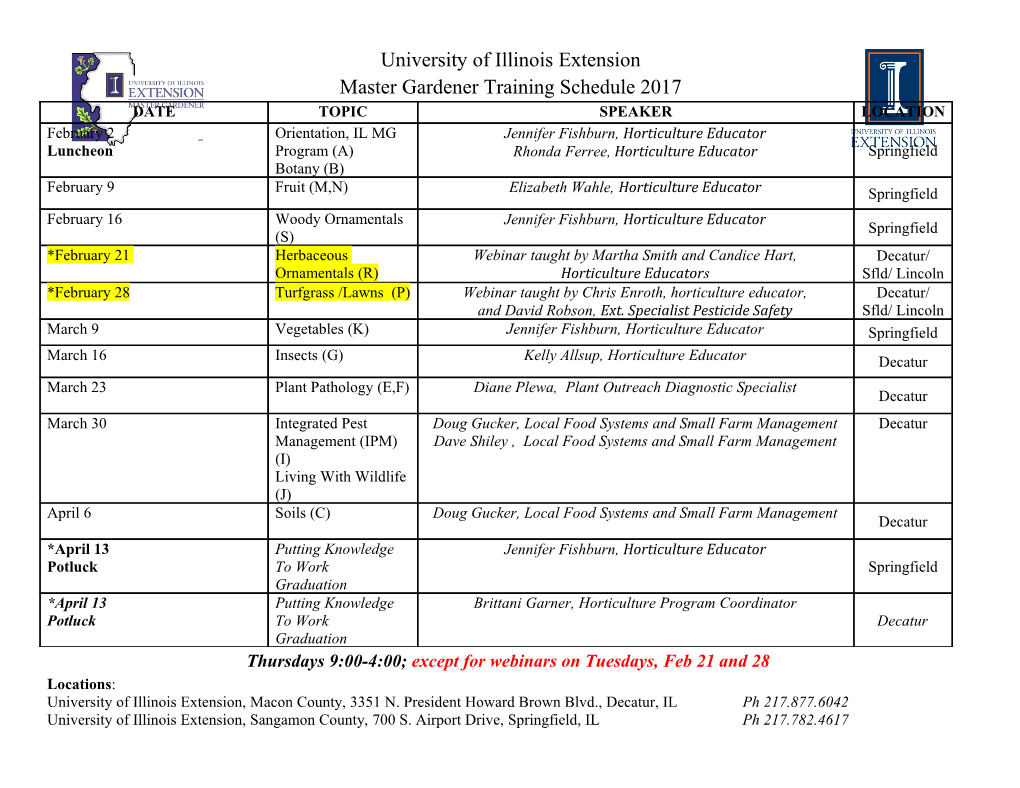
Contrasting size evolution in marine and freshwater diatoms E. Litchmana,b,1, C. A. Klausmeiera,c, and K. Yoshiyamaa,d aKellogg Biological Station, Michigan State University, Hickory Corners, MI 49060; bZoology Department, Michigan State University, East Lansing, MI 48824; cPlant Biology Department, Michigan State University, East Lansing, MI 48824; and dDepartment of Chemical Oceanography, Ocean Research Institute, University of Tokyo, Tokyo 164-8639, Japan Edited by Simon A. Levin, Princeton University, Princeton, NJ, and approved December 8, 2008 (received for review October 28, 2008) Diatoms are key players in the global carbon cycle and most aquatic Marine and freshwater environments provide an opportunity ecosystems. Their cell sizes impact carbon sequestration and en- for such a comparison, because they differ in many physical and ergy transfer to higher trophic levels. We report fundamental chemical characteristics that may exert contrasting selective differences in size distributions of marine and freshwater diatoms, pressures on phytoplankton cell size. Although both nitrogen with marine diatoms significantly larger than freshwater species. (N) and phosphorus (P) can limit different species at different An evolutionary game theoretical model with empirical allometries times and locations, overall, N is thought to be more often of growth and nutrient uptake shows that these differences can be limiting than P in marine systems, with the reverse in freshwaters explained by nitrogen versus phosphorus limitation, nutrient fluc- (9). The mixed layer depth (MLD) is typically greater in marine tuations and mixed layer depth differences. Constant and pulsed systems than in freshwaters (10–12), thus affecting diatom phosphorus supply select for small sizes, as does constant nitrogen persistence in the water column. Consequently, we anticipate supply. In contrast, intermediate frequency nitrogen pulses com- differences in phytoplankton (diatom) size spectra between mon in the ocean select for large sizes or the evolutionarily stable marine and freshwater systems. No studies to date have com- coexistence of large and small sizes. Size-dependent sinking inter- pared diatom size distributions from the 2 environments. acts with mixed layer depth (MLD) to further modulate optimal sizes, with smaller sizes selected for by strong sinking and shallow Diatom Size Distributions in Marine and Freshwaters. We compiled ECOLOGY MLD. In freshwaters, widespread phosphorus limitation, together data on diatom cell sizes from diverse marine and freshwater with strong sinking and shallow MLD produce size distributions ecosystems, including temperate and polar oceans and North with smaller range, means and upper values, compared with the American, European, and Japanese lakes (see Methods). We find ocean. Shifting patterns of nutrient limitation and mixing may alter that marine and freshwater diatom size distributions are signif- icantly different (Fig. 1). Marine diatoms span almost 9 orders diatom size distributions, affecting global carbon cycle and the of magnitude in cell volume, with the largest species reaching structure and functioning of aquatic ecosystems. Ͼ109 m3, whereas cell volumes of freshwater diatoms vary Ͻ6 orders of magnitude, with the largest cells Ϸ106 m3. Mean cell evolutionarily stable strategy ͉ phytoplankton ͉ ͉ volumes of marine diatoms are an order of magnitude greater resource competition resource fluctuations than in freshwaters (Fig. 1). The differences in size distributions between marine and freshwater diatoms that we report here ody size is one of the most fundamental traits of organisms, appear universal, because they are robust across diverse ecosys- Baffecting almost all aspects of their physiology and ecology tems (Fig. 1), and thus suggest fundamentally different selective (1, 2). Consequently, it is a major component of fitness subject pressures in marine versus freshwater realm. What leads to the to evolution by natural selection (3, 4). Macroevolutionary and contrasting size distributions between marine and freshwater ecological questions about body size include the direction of environments? long-term size evolution, the maintenance of size diversity, and Small cell size makes the acquisition of limiting nutrients more how body size is shaped by environmental factors. Phytoplank- efficient because of high surface area to volume ratio and, ton have been used as a model system to explore many funda- should, therefore, be competitively advantageous under nutrient mental questions in ecology (5). Here, we investigate the role of limitation (13). High resource concentrations select species with environmental drivers on size evolution in a major group of high maximum growth rates (14), and because maximum growth phytoplankton, diatoms. rates are negatively correlated with cell size (15), small species Diatoms are ubiquitous in both marine and freshwater envi- should again have a competitive advantage. Why then do large ronments, contributing up to 25% of the world’s primary pro- sizes ever evolve? One potential mechanism that has been ductivity and forming the basis of many aquatic food webs (6). hypothesized to select for large-celled species is nutrient storage Diatom size distributions greatly influence carbon sequestration ability in a fluctuating nutrient environment (16, 17). efficiency: due to their faster sinking and slower dissolution, In phytoplankton, key parameters of nutrient-dependent large cells export disproportionately large amount of carbon to growth and uptake scale allometrically with cell volume (16, 18). the ocean floor (6, 7). Cell sizes of diatoms and other phyto- Consequently, selective pressures that lead to the evolution of plankton determine the flow of energy and materials to higher nutrient acquisition traits (e.g., nutrient limitation) will likely trophic levels and, hence, the structure and functioning of aquatic food webs (6). Consequently, understanding the factors Author contributions: E.L. and C.A.K. designed research; E.L., C.A.K., and K.Y. performed that drive cell size evolution is needed to predict global carbon research; C.A.K. contributed new reagents/analytic tools; E.L., C.A.K., and K.Y. analyzed cycling and the functioning of diverse aquatic ecosystems. Phy- data; and E.L., C.A.K., and K.Y. wrote the paper. toplankton (diatom) cell size is a result of diverse selective forces The authors declare no conflict of interest. present in the environment, such as different patterns of nutrient This article is a PNAS Direct Submission. limitation and physical mixing, and grazing pressure (8). Com- 1To whom correspondence should be addressed. E-mail: [email protected]. paring size distributions across ecosystems that differ in their This article contains supporting information online at www.pnas.org/cgi/content/full/ selective pressures should provide new insights on cell size 0810891106/DCSupplemental. evolution. © 2009 by The National Academy of Sciences of the USA www.pnas.org͞cgi͞doi͞10.1073͞pnas.0810891106 PNAS Early Edition ͉ 1of6 Downloaded by guest on September 25, 2021 10 marine vs. freshwater p<0.0001 The following species-specific parameters are assumed to A hi depend allometrically on cell size s: Qmin, Qmax, V max, ϱ, K, and v. Other parameters are size- and species-independent, except 8 lo lo ) ϭ Ϫ ϭ 3 AB BB V max, which is set to V max ϱ(Qmax Qmin) so that Q Qmax m hi µ when growing at maximum growth rate (16, 21). V max is con- lo 6 C strained to be less than or equal to Vmax, but we obtain similar CD D D results without this constraint. Nutrients are continuously taken up by phytoplankton and cell volume ( 4 10 periodically resupplied with period T (day). Between mixing Log events, 2 dR ϭϪͩV hi Ϫ ͑ V hi dt max,i max,i i 0 Pacific Mediter Barents Baltic Finnish lakes Biwa Crater L. Madison Ϫ Qi Qmin,i R Ϫ V lo ͒ ͪ N Fig. 1. Box-Whisker plot of log10 cell volume distributions of diatoms max,i Ϫ ϩ i Qmax,i Qmin,i R Ki occurring in diverse marine and freshwater environments. Shaded distribu- tions are from marine and clear distributions are from freshwater environ- Mixing events replace a fraction a of the mixed layer with water ments. Cell volumes are measured in cubic micrometers. The distributions are from the deep, which is assumed to contain no phytoplankton arranged in order of decreasing means (not medians) and do not account for but abundant nutrients Rin. Thus, species abundance. The data on cell volumes were log10-transformed and compared using nonparameteric Wilcoxon test (pooled marine vs. freshwater ͑ ϩ͒ ϭ ͑ Ϫ ͒ ͑ Ϫ͒ ϩ environments) and Tukey-Kramer HSD test (each ecosystem, square root R jT 1 a Ri jT aRin transformed log10 volumes) using JMP (SAS). See Methods for sources of data ͑ ϩ͒ ϭ ͑ Ϫ ͒ ͑ Ϫ͒ and statistical details. Number of species for each ecosystem is given in Ni jT 1 a Ni jT parentheses: Pacific Ocean (n ϭ 76), Mediterranean Sea (Bay of Marseille) (n ϭ 103), Barents Sea (n ϭ 167), Baltic Sea (n ϭ 220), Finnish lakes (n ϭ 329), Lake for all positive integers j. Biwa, Japan (n ϭ 31), Crater Lake, OR (n ϭ 56) and Madison, WI area lakes (n ϭ We analyze the model using techniques from evolutionary 72). Baltic Sea data include some freshwater species and Finnish lakes data game theory (19, 23). We take log10 cell volume, s, as our trait. include some marine species (possibly from coastal lakes). A central concept in this approach is invasion fitness, g(sinv,ជsres), which is defined as the invasion rate of a new strategy, sinv, invading an established community, ជsres, when rare. Because alter phytoplankton cell size. Using techniques from evolution- our model has both periodic forcing and physiologically struc- ary game theory to integrate these physiological traits into fitness tured populations, we numerically calculate invasion fitness as (5, 19), we derive evolutionarily stable cell sizes of marine and follows: (i) we solve for the resident community dynamics until freshwater diatoms under different scenarios of nutrient limita- it reaches a stable limit cycle; (ii) we force the invader’s quota tion, including fluctuating nutrient conditions.
Details
-
File Typepdf
-
Upload Time-
-
Content LanguagesEnglish
-
Upload UserAnonymous/Not logged-in
-
File Pages6 Page
-
File Size-