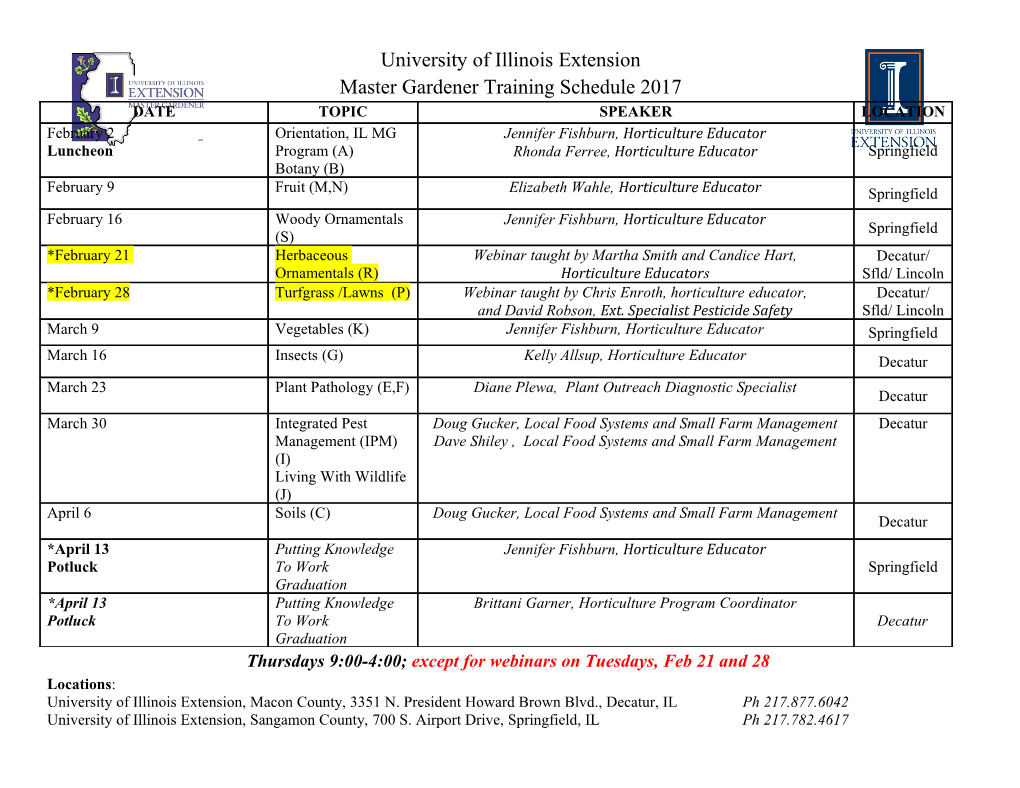
Physiological, biochemical and behavioural responses of freshwater crayfish (Paranephrops zealandicus) to hypoxia and water nutrients A thesis submitted in partial fulfilment of the degree of Master of Science in Zoology at the University of Canterbury by Rebecca Broughton University of Canterbury 2015 1 Table of Contents Abstract 9 1. General introduction 12 1.1. Context 12 1.1.1. Eutrophication: Causes and prevalence 12 1.1.2. Hypoxia: Causes and impacts 15 1.1.3. New Zealand freshwater crayfish 17 1.2. Background 18 1.2.1. Physiological responses of freshwater crayfish to environmental stressors 18 1.2.2. Biochemical responses of freshwater crayfish to hypoxia and high nutrients 20 1.2.3. Behavioural responses of freshwater crayfish to hypoxia 22 1.3. Previous physiological research on Paranephrops zealandicus 25 1.4. Aims 25 2. General methods 28 2.1. Collection and maintenance 28 2.2. Metabolic rate 28 2.2.1. Respirometry types 30 2.3. Ammonia and nitrite 31 2.4. Measurement of heart rate 32 2.5. Haemolymph collection, analysis and storage 33 2.5.1. Haemolymph CO2, pH, and PO2 samples 33 2.5.2. Tissue collection and storage 34 2.6. Biochemical analysis of tissues 34 2.6.1. Tissue preparation 34 2.6.2. Tissue analysis 35 3. Oxygen consumption and heart rate responses of Paranephrops zealandicus to hypoxia and increased nutrients 38 3.1. Introduction 38 3.2. Methods and Materials 41 2 3.2.1. Physiological responses to hypoxia under progressive closed box respirometry 41 3.2.2. Physiological responses to hypoxia under semi-closed box respirometry 42 3.2.3. Physiological responses to hypoxia and/or high nutrients under prolonged flow-through respirometry 42 3.2.4. Data analysis and statistical methods 43 3.3. Results 44 3.3.1. Physiological responses to hypoxia under progressive closed box respiromentry 44 3.3.2. Physiological responses to hypoxia under semi closed box respirometry 52 3.3.3. Physiological responses to hypoxia and/or high nutrients under prolonged flow -through respirometry 54 3.4. Discussion 60 3.4.1. Physiological responses to hypoxia- oxygen consumption 61 3.4.2. Physiological responses to hypoxia- heart rate 63 3.4.3. Physiological responses to severe hypoxia and high nutrients- nitrite 66 3.4.4. Physiological responses to severe hypoxia and high nutrients- ammonia 67 3.5. Summary 68 4. Biochemical responses of Paranephrops zealandicus to hypoxia and increased nutrients 71 4.1. Introduction 71 4.2. Methods and Materials 73 4.2.1. Haemolymph parameters 73 4.2.2. Glucose and lactate concentrations 74 4.2.3. Data analysis and statistical methods 75 4.3. Results 76 4.3.1. Haemolymph parameters 76 4.3.2. Lactate and Glucose 78 4.4. Discussion 82 4.4.1. Haemolymph gases 82 4.4.2. Lactate 84 4.4.3. Glucose 85 4.5. Summary 88 5. Behavioural responses of freshwater crayfish Paranephrops zealandicus to hypoxia. 90 5.1. Introduction 90 3 5.2. Methods and materials 92 5.2.1. Avoidance test chamber 93 5.2.2. Experimental design of avoidance experiments 96 5.2.3. Emersion test chamber 98 5.2.4. Experimental design of emersion experiments 99 5.2.5. Data analysis and statistical methods 100 5.3. Results 100 5.3.1. Avoidance 100 5.3.2. Emergence 102 5.4. Discussion 104 5.4.1. Hypoxia avoidance 104 5.4.2. Emergence 106 5.5. Summary 109 6. General discussion 111 6.1. Aims and background 111 6.2. Physiological responses to hypoxia 111 6.2.1. Oxygen consumption responses to hypoxia 111 6.2.2. Heart rate responses to hypoxia 112 6.3. Biochemical responses to hypoxia 113 6.3.1. Haemolymph responses to hypoxia 113 6.3.2. Lactate responses to hypoxia 113 6.3.3. Glucose responses to hypoxia 114 6.3.4. Responses to nutrients 114 6.4. Behavioural responses 115 6.4.1. Avoidance 115 6.4.2. Emergence 115 6.5. Methodological considerations and issues highlighted for further study 116 6.5.1. Variability in responses 117 6.5.2. Behavioural methodologies 118 6.5.3. Future studies 119 Acknowledgements 122 References 124 Appendix 158 4 List of Figures Figure 2.1: Photograph of the respirometer 29 Figure 2.2: Diagrammatical representation of the respirometer 31 Figure 2.3: Photograph of the infrared sensor used in experiments sensor 32 Figure 3.1: Oxygen consumption values of P. zealandicus during progressive hypoxia 45 Figure 3.2: Raw oxygen consumption values of P. zealandicus during progressive hypoxia 46 Figure 3.3: Oxygen consumption patterns of individual P. zealandicus during progressive hypoxia 47 Figure 3.4: “Brokenstick” analysis of PCRIT using raw oxygen consumption values of P. zealandicus during progressive hypoxia 48 Figure 3.5: “Brokenstick” analysis of PCRIT using grouped oxygen consumption values of P. zealandicus during progressive hypoxia 49 Figure 3.6: Graphical analysis of PCRIT using raw oxygen consumption values of P. zealandicus during progressive hypoxia 50 Figure 3.7: Graphical analysis of PCRIT using grouped oxygen consumption values of P. zealandicus during progressive hypoxia 50 Figure 3.8: Raw heart rate values of P. zealandicus under progressive hypoxia 51 Figure 3.9: Heart rate values of P. zealandicus under progressive hypoxia 52 Figure 3.10: The effect of respirometry type on the oxygen consumption of P. zealandicus 53 Figure 3.11: Heart rate values of P. zealandicus comparing semi and closed-box respirometry 54 5 Figure 3.12: Oxygen consumption values for every hour of P. zealandicus over a six hour period at 155 mmHg 55 Figure 3.13: Oxygen consumption values for every hour of P. zealandicus over a six hour period at 30 mmHg 56 Figure 3.14: Oxygen consumption values for every hour of P. zealandicus over a six hour period at 10 mmHg 56 Figure 3.15: Oxygen consumption values for every hour of P. zealandicus over a six hour period at 10 mmHg and 20 mg mL-1 of sodium nitrite 57 Figure 3.16: Oxygen consumption values for every hour of P. zealandicus over a six hour period at 10 mmHg and 30 mg mL-1 of ammonia chloride 57 Figure 3.17: Oxygen consumption values of P. zealandicus over the six hours of each treatment type 58 Figure 3.18: Heart rate values for every hour of P. zealandicus over a six hour period of all treatment 59 Figure 3.19: Heart rate values of P. zealandicus over the six hours of each treatment type 60 Figure 4.1: The effect of PO2 and nutrients on the haemolymph PO2 of P. zealandicus 76 Figure 4.2: The effect of water partial oxygen concentration and nutrients on the haemolymph pH of P. zealandicus 77 Figure 4.3: The effect of water partial oxygen concentration and nutrients on the haemolymph partial carbon dioxide concentration of P. zealandicus 78 Figure 4.4: The effect of water partial oxygen concentration and nutrients on the haemolymph lactate of P. zealandicus 79 Figure 4.5: The effect of water partial oxygen concentration and nutrients on the haemolymph glucose of P. zealandicus 80 6 Figure 4.6: The effect of water partial oxygen concentration and nutrients on the tail muscle lactate of P. zealandicus 81 Figure 4.7: The effect of water partial oxygen concentration and nutrients on the tail muscle glucose of P. zealandicus 81 Figure 5.1: Diagrammatical representation of the avoidance chamber apparatus 94 Figure 5.2: Diagrammatical representation of the avoidance chamber apparatus. 95 Figure 5.3: Photograph showing the dye test and distinct separation of recti-linear flows on each side. 95 Figure 5.4: Diagrammatical representation of the emergence chamber apparatus. 98 Figure 5.5: The effect of side on the avoidance choice of P. zealandicus 101 Figure 5.6: The effect of water partial oxygen concentration combinations on the avoidance choice of P. zealandicus 101 Figure 5.7: Rate of water partial oxygen concentration decline during the progressive hypoxia emergence experiment 103 Figure 5.8: The time of emersion and partial water oxygen concentration of P. zealandicus under progressive, intermediate and extreme hypoxia 103 7 List of Tables Page Table 3.1 The weights and sample values of P. zealandicus used in the 43 measurement of MO2 and heart rate under prolonged exposures Table 4.1 The weights and sample values of P. zealandicus used in the 75 measurements of lactate and glucose concentrations Table 5.1 The experimental protocols used in the avoidance experiments 97 8 Abstract Hypoxia is a decline in water oxygen levels and is often caused by aquatic nutrient enrichment. It is recognised as one of the foremost environmental issues impacting and influencing species distribution. This population level effect is likely mediated by the physiological, biochemical and behavioural impacts associated with reduced oxygen. Most aquatic animals are able to maintain a constant oxygen uptake with small reductions in water oxygen, and are thus able to fuel the various processes driven by aerobic metabolism. However, as hypoxia persists or strengthens, regulation fails and aerobic metabolism is impaired leading to impacts such as the loss of acid-base homeostasis. The nutrient loading that often drives hypoxic episodes (through increased primary production) may impose additional stress that could also impact hypoxic responses and tolerance. The New Zealand freshwater crayfish Paranephrops zealandicus often inhabits streams that are in close proximity to farmland and are therefore prone to agricultural and pastoral run-off events. Consequently, crayfish will be faced with the challenge of energy homeostasis in conditions where aerobic capacity is compromised. The current study has investigated the physiological, biochemical and behavioural responses of P.
Details
-
File Typepdf
-
Upload Time-
-
Content LanguagesEnglish
-
Upload UserAnonymous/Not logged-in
-
File Pages158 Page
-
File Size-