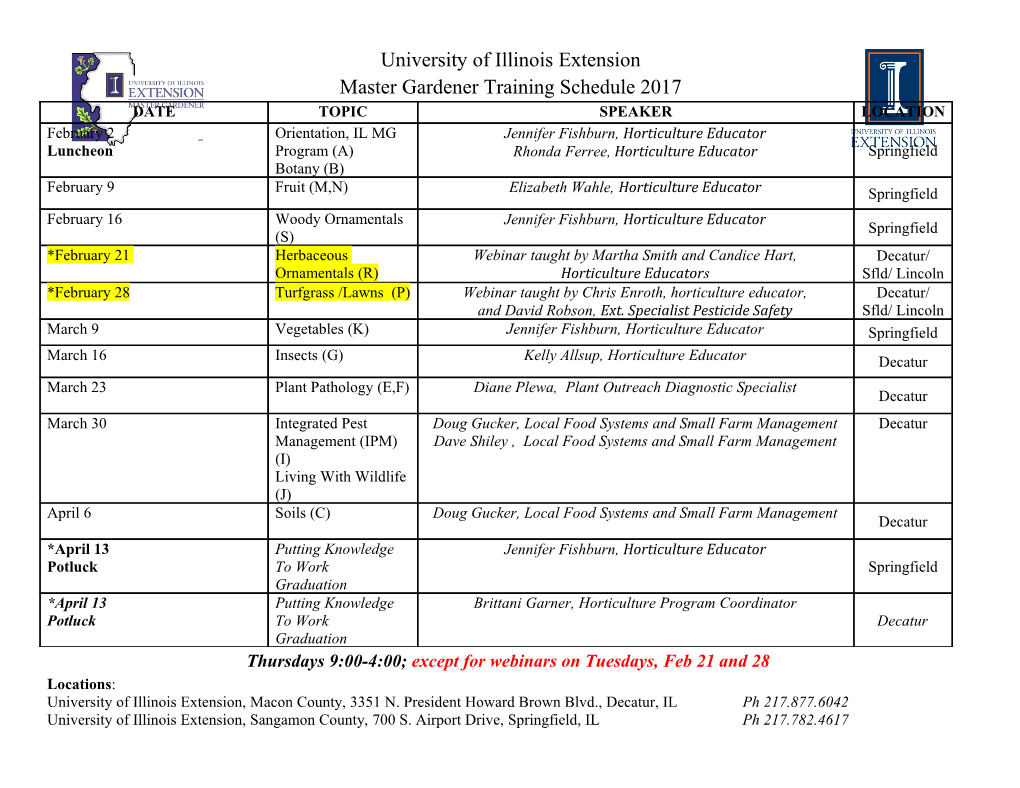
MICROWAVE CAVITY METHOD FOR MEASURING PLASMA PROPERTIES APPROVED: J&jor Professor Minor Professor (j Directoj^of the Dep^frtirient of Physics Dean of the Graduate School MICROWAVE CAVITY METHOD FOR MEASURING PLASMA PROPERTIES THESIS Presented to the Graduate Council of the North Texas State University in Partial Fulfillment of the Requirements For the Degree of MASTER OF SCIENCE By Ronald H. Freeman, B.S, Denton, Texas August, 1969 TABLE OF CONTENTS LIST OF TABLES iv LIST OF ILLUSTRATIONS V Chapter I. INTRODUCTION 1 II. THEORY, DESIGN AND OPERATIONAL PROCEDURES. 16 III. ANALYSIS AND CONCLUSIONS 28 APPENDIX . 37 BIBLIOGRAPHY 59 in LIST OF TABLES Table Page I. Resonant Frequency shift versus Plasma Discharge Current Data for Argon at 200 Microns Pressure.... 55 II. Resonant Frequency shift versus Plasma Discharge Current Data for Argon at 277 Microns Pressure.... 55 III. Resonant Frequency shift versus Plasma Discharge Current Data for Argon at 267.5 Microns Pressure.. 56 IV. Resonant Frequency shift versus Plasma Discharge Current Data for Argon at 126.4 Microns Pressure.. 56 V. Resonant Frequency shift versus Plasma Discharge Current Data for Argon at 514 Microns Pressure.... 57 VI. Resonant Frequency shift versus Plasma Discharge Current Data for C>2 at 250 Microns Pressure 57 VII. Resonant Frequency shift versus Plasma Discharge Current Data for C>2 at 200 Microns Pressure 58 VIII. Resonant Frequency shift versus Plasma Discharge Current Data for at 95 Microns Pressure 5 8 IX. Resonant Frequency shift versus Plasma Discharge Current Data for 02 at 210 Microns Pressure 5 8 IV LIST OF ILLUSTRATIONS Figure Page 1. Hypersonic Flow Patterns or Reentry Flow-Field Plasmas • 3 8 2. Basic Electromagnetic Wave-Plasma Interaction. ... 39 3. Block Diagram of Experimental Equipment 40 4. Cylindrical Cavity 41 5. Mode Patterns for the TMn1f) Mode and Direction of the Electric Field 42 6. Resonance Line Shape 43 7. Standing-Wave Ratios, in the Feed Line for (A) an Empty and (B) a Plasma-Filled Resonant Cavity in the Vicinity of Resonance, Showing Detuning. .... 44 8. Block Diagram for Discharge Tube 45 9. Electron Discharge Tube 46 10. Resonant Frequency Shift vs Discharge Current for Argon at p=200 Microns 47 11. Resonant Frequency Shift vs Discharge Current for Argon at p=277 Microns 48 12. Resonant Frequency Shift vs Discharge Current for Argon at p=267 Microns 49 13. Resonant Frequency Shift vs Discharge Current for Argon at "p=126.4 Microns . 50 14. Resonant Frequency Shift vs Discharge Current for Argon at p=514 Microns 51 15. Resonant Frequency Shift vs Discharge Current for at p=250 Microns. 52 16. Resonant Frequency Shift vs Discharge Current for O2 at p=200 Microns 53 v Figure Page 17. Resonant Frequency Shift vs Discharge Current for C>2 at p=9 5 Microns 5 4 18. Resonant Frequency Shift vs Discharge Current for 02 at p=210 Microns , 54 VI CHAPTER I INTRODUCTION The plasma sheath which envelops a spacecraft during entry into an atmosphere disrupts electromagnetic prop- agation links to and from the spacecraft. The resultant entry communication problem has faced mission planners since velocities greater than Mach 10 were first achieved. Researchers in many cases have been able to design around this radio blackout problem but not without the loss of valuable research and operational data or the requirement of elaborate data storage and playback systems and recovery packages. This problem is of much greater concern for advanced missions involving manned maneuverable spacecraft reentries, and for scientific entry probes to other planets such as Mars or Ventis, both of which require real-time communication links. This discussion is concerned primarily with communica- tions blackout during spacecraft entry into a planetary atmosphere. The gas in the shock layer, between shock wave and vehicle surface, ionizes from the intense heating which takes place in the bow shock wave and a viscous region of high gas enthalpy. This ionization may persist throughout the subsequent flow over the vehicle and into the wake, thus completely engulfing the vehicle and its communica- tions elements. This layer contains a high concentration of free electrons. The schematic representation of continuous flow-field regions.near typical reentry bodies illustrates the fact that several distinctly different types of plasma may be present,as shown in Fig. 1. The outer region (between the shock wave and viscous layers) consists of inviscid air which is first compressed and heated by a strong bow shock wave. During this time many free electrons are produced. When this fluid expands around the vehicle, some of the electrons and ions recombine, but in general, this flow is not one of complete chemical equilibrium. (4,6) There is a viscous boundary-layer region close to the body surface where fluid is decelerated from the inviscid flow velocity to zero velocity at the surface. There may be additional electron production within the layer, or there may be primarily recombination, depending upon the aero- dynamics involved. Also, because of the ablation of surface material during reentry, this fluid may be a reacting mixture of air and ablation products. As in the case of the inviscid plasma, the boundary-layer plasma is generally not in equilibrium. The inner plasma region(between the free-shear layer and the rear-body surfaces) is one of viscous separated fluid^which is composed of boundary-layer air "contaminated" with ablation material from the heat shield. This separated mixture recirculates in the base-flow region and is finally scavenged into the wake. Because of the long dwell time of this fluid, the separated plasma is likely to approach equilibrium. The signal propagation* from an antenna on the body will be through both the inviscid plasma and one of the viscous plasma regions(depending on the reentry dynamics or programmed maneuvers by the onboard computer or the manual commands by the astronaut, the aspect angle, attitude, and roll orientation of the reentry vehicle) and will never be through a viscous region only, or the inviscid region only. Since the general problem involves propagation through all these plasmas, it is necessary to determine the electron concentration in each type of plasma. Before entering into this discussion of the electron concentration, it is advantageous to gain insight into the manner by which these free electrons can disrupt electro- magnetic propagation by reflection and attenuation of these electromagnetic waves. (3) First, assume that a plasma contains a like number of positively and negatively charged particles and can be considered macroscopically neutral. Most of the negative charges are free electrons and most of the ions are positive in a reentry plasma. On a microscopic scale, these charged particles maintain an average separation due to their electrostatic fields, which, for simplicity, can be considered as the equilibrium spacing. If for some reason one of the charges is perturbed out of equilibrium configuration and the other charges remain fixed, the dynamic picture would be an oscillation of the single charge about its equilibrium position, like that of a spring and mass oscillator. The particle represents the mass, the restoring electrostatic force due to neighboring charges represents the spring, and collision of the- charge with other particles in the plasma represents the damping force. The meaning of "plasma frequency" now becomes clear; it is the characteristic or natural oscillation frequency of a charge in the plasma. Electrons will oscillate at a value, given as follows:(1) 2 f = (—®—N£)^^ 8970 (Nq)^ (i) u me where me is the electron mass, e is the electron charge, Ne is the number of free electrons in a cubic centimeter, and f is the plasma frequency in cycles per second. Since P me and e are constants, the value of plasma frequency depends only on the electron concentration, which deter- mines the restoring force. The damping parameter for electron oscillation, called collision frequency, v, can be expressed by the following equation: (3) v - c £ n. Q • (2) e i. 11 • The mean thermal electron velocity cQ takes the following equation form: S = (SI)15 •. <3) . e urne The expression for collision frequency is evaluated by sxamming the product of concentration, ri^, and the velocity- averaged collision cross-section, Q^, for all species in the plasma that the electron can collide with. These include both neutral species and ions. Therefore, the dynamic picture describes the electron being perturbed out of position and then allowed to oscillate in accordance with forces from within the plasma. In the case of electromagnetic waves interacting, however, there is a continu- ous periodic driving force on the electron, due to the interaction between the electric field of. the wave and the electrostatic field of the electrons. This is illustrated in Fig. (2) to help visualize the problem. Part (a) denotes a driving frequency considerably less than the natural- frequency of the plasma electron,and for low collisional damping. In part (b) the electron thus appears to have little inertia and will closely follow the driving or signal frequency, f. Since the oscillating charge will radiate (due to charge acceleration) as a dipole radiator, a forward and a backward electromagnetic wave will occur (part c). The backward wave is seen as the reflected wave in free space, whereas the forward wave largely cancels the signal resulting in a greatly weakened or'attenuated wave in the propagation direction. This process is repeated is each unit thickness of plasma so that wave attenuation increases directly with the plasma thickness. The amount of reflection seen in free space increases with plasma thickness to a thickness comparable to wavelength of signal in the plasma. The amount of reflection for larger thickness does not increase because the backward wave in the plasma is attenuated.
Details
-
File Typepdf
-
Upload Time-
-
Content LanguagesEnglish
-
Upload UserAnonymous/Not logged-in
-
File Pages67 Page
-
File Size-