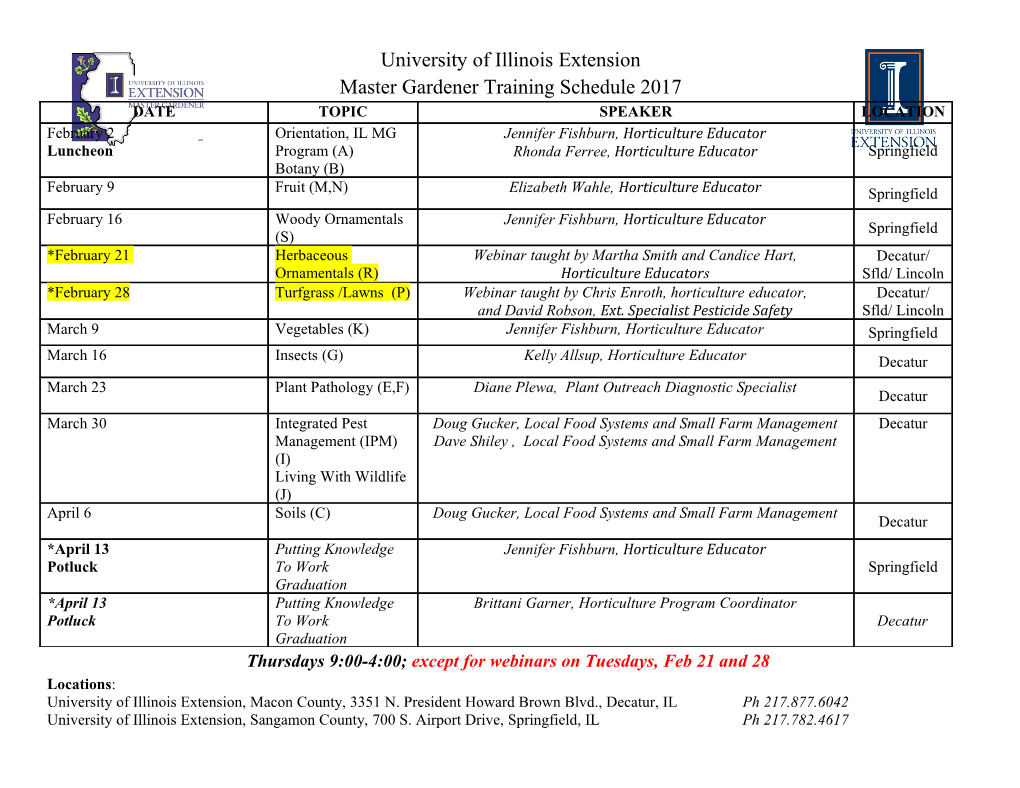
NANOSCALE ENGINEERING FOR THE DESIGN OF EFFICIENT INORGANIC-ORGANIC HYBRID THERMOELECTRICS A Dissertation by LANCE ROBERT BROCKWAY Submitted to the Office of Graduate and Professional Studies of Texas A&M University in partial fulfillment of the requirements for the degree of DOCTOR OF PHILOSOPHY Chair of Committee, Sreeram Vaddiraju Committee Members, Rusty Harris Tahir Cagin Mustafa Akbulut Head of Department, Nazmul Karim May 2014 Major Subject: Chemical Engineering Copyright 2014 Lance Robert Brockway ABSTRACT Research aimed at enhancing the thermoelectric performance of semiconductors comprised of only earth-abundant elements has recently come under renewed focus as these materials systems offer a cost-effective path for scavenging waste heat. In light of the prediction that nanostructuring could increase the thermoelectric performance of materials; semiconductor nanowires comprised of non-toxic, low cost, and earth- abundant elements were synthesized and studied for their thermoelectric performance. For accomplishing this task, zinc phosphide (Zn3P2), zinc antimonide (Zn4Sb3), and zinc oxide (ZnO) nanowires were synthesized on the gram-quantity scale. Post-synthesis decomposition techniques were developed to controllably reduce the nanowire diameter and to create nanotubes. This decrease in nanowire diameter comes at an additional cost of an exponential decrease in surface stability. To combat this, a vapor phase surface passivation technique was developed to protect the nanowire surfaces from degradation and agglomeration. Finally, gram quantities of both functionalized and unfunctionalized nanowires were compressed into dense nanobulk pellets and characterized for their thermoelectric performance. The reactive vapor transport synthesis technique resulted in gram-quantities of single-crystalline nanowires with consistent 20 nm diameters. The nanowire diameters were further reduced to create sub-5 nm quantum wires and nanotubes using controlled decomposition. A self-consistent mechanism to describe this phenomenon was proposed. The nanowires were further surface-functionalized with various organic molecules to ii prevent surface degradation and to control the interfacial transport properties within the consolidated nanowire-pellets. The stability enhancement of the nanowires using this vapor-phase self-assembled monolayer technique was shown using traditional organic characterization techniques and suspension stability. Zn3P2 and ZnO nanowires were then hot-pressed and spark plasma sintered, respectively, into nanobulk pellets. It was observed that the nanowires in the Zn3P2 pellet did not break upon compaction, but bent elastically to achieve their sintered density; this was confirmed using a single nanowire inside a TEM. The thermoelectric performance of the functionalized nanowires was shown to be 3-fold higher than that of unfunctionalized nanowires due to less nanowire surface oxidation. Finally, the ZnO nanowire-bulk pellets were optimally-doped resulting in a 30% decrease in thermal conductivity compared to the bulk and the highest reported n-type oxide zT to date of 0.60. iii ACKNOWLEDGEMENTS I would like to thank the Vaddiraju research group for all of their help, guidance, and support throughout this research project including, but not limited to, Sreeram Vaddiraju, Yongmin Kang, Venkata Vasiraju, and Maxime Van Laer. Additionally I would like to thank Jean-Pierre Fleurial and Sabah Bux of the NASA Jet Propulsion Laboratory for their generosity in helping with all of the thermoelectric materials characterization and facilities use. Also thanks to Jacek Jasinski of the Conn Center for Renewable Energy at the University of Louisville for his help with the transmission electron microscopy and data analysis. Finally, I would like to thank my family for their encouragement throughout the entirety of this project. iv NOMENCLATURE 4-ATP 4-Aminothiophenol 3DT 3-Propanedithiol BDT p-Benzenedithiol COD Crystallography Open Database Cp Heat Capacity CVD Chemical Vapor Deposition D Diameter D0 Initial Diameter DSC Differential Scanning Calorimetry EB Energy Barrier EDS Energy Dispersive Spectroscopy Eg Bandgap FFT Fast Fourier Transform FTIR Fourier-transform Infrared Spectroscopy h Planck’s Constant HR High Resolution kB Boltzmann’s Constant L Lorenz Number LFA Laser Flash Analyzer me Theoretical Electron Mass v m* Effective Electron Mass n Carrier Concentration NW Nanowire P Pressure S Seebeck Coefficient SAED Small Angle Electron Diffraction SAM Self-assembled Monolayer SEM Scanning Electron Microscopy SLV Solid-vapor-liquid SPS Spark Plasma Sintering STEM Scanning Transmission Electron Microscopy STM Scanning Tunneling Microscopy t Time T Temperature TE Thermoelectric TEG Thermoelectric Generator TEM Transmission Electron Microscopy THF Tetrahydrofuran UV-Vis Ultra-violet/Visible Spectroscopy VLS Vapor-liquid-solid XPS X-ray Photoelectron Spectroscopy XRD X-ray Diffraction vi zT Dimensionless Thermoelectric Figure of Merit α Absorption Coefficient α Thermal Diffusivity Δ Lattice mismatch η Reduced Fermi-level κ Total Thermal Conductivity κl Lattice Thermal Conductivity κe Electronic Thermal Conductivity μ Hall Mobility ν Frequency ρ Electrical Resistivity ρ Density σ Electrical Conductivity vii TABLE OF CONTENTS Page ABSTRACT .......................................................................................................................ii ACKNOWLEDGEMENTS .............................................................................................. iv NOMENCLATURE ........................................................................................................... v TABLE OF CONTENTS ............................................................................................... viii LIST OF FIGURES ........................................................................................................... xi LIST OF TABLES .........................................................................................................xvii CHAPTER I INTRODUCTION ....................................................................................... 1 CHAPTER II LITERATURE AND BACKGROUND .................................................... 6 Waste Heat Recovery Using Thermoelectrics ............................................................... 6 Nanowire Thermoelectrics ............................................................................................. 8 Selection of Materials..................................................................................................... 9 Nanowire Synthesis and Diameter Control .................................................................. 11 Surface Passivation ...................................................................................................... 12 Nanobulk Materials ...................................................................................................... 13 CHAPTER III NANOWIRE SYNTHESIS AND CHARACTERIZATION METHODS ....................................................................................................................... 15 Introduction .................................................................................................................. 15 Nanowire Synthesis Methods ....................................................................................... 16 GaN Nanowire Synthesis ......................................................................................... 16 II-V Nanowire Synthesis .......................................................................................... 18 Nanowire Characterization Techniques ....................................................................... 21 Nanowire Consolidation Techniques ........................................................................... 22 Thermoelectric Performance Characterization............................................................. 23 CHAPTER IV POST-SYNTHESIS FORMATION OF QUANTUM WIRES .............. 24 Introduction .................................................................................................................. 24 Decomposition Methods .............................................................................................. 26 Results .......................................................................................................................... 27 viii Nanowire Synthesis .................................................................................................. 27 Nanowire Diameter Reduction ................................................................................. 28 Decomposition Mechanism and Kinetics ................................................................. 30 In situ TEM Decomposition ..................................................................................... 39 Bandgap Blue-Shift .................................................................................................. 40 Conclusion .................................................................................................................... 43 CHAPTER V POST-SYNTHESIS NANOTUBE FORMATION ................................. 44 Introduction .................................................................................................................. 44 Decomposition Methods .............................................................................................. 45 Results .........................................................................................................................
Details
-
File Typepdf
-
Upload Time-
-
Content LanguagesEnglish
-
Upload UserAnonymous/Not logged-in
-
File Pages143 Page
-
File Size-