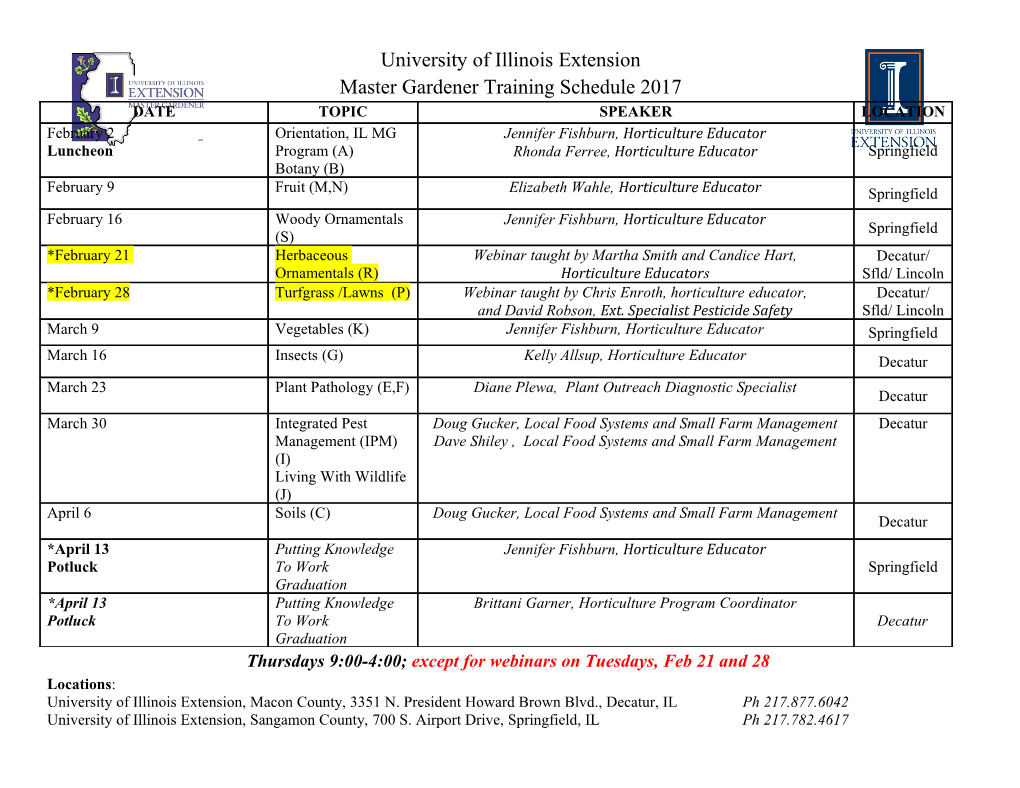
https://doi.org/10.1130/G48461.1 Manuscript received 24 April 2020 Revised manuscript received 30 November 2020 Manuscript accepted 3 December 2020 © 2021 The Authors. Gold Open Access: This paper is published under the terms of the CC-BY license. Published online 5 February 2021 Is the Eastern Denali fault still active? Minhee Choi, David W. Eaton and Eva Enkelmann Department of Geoscience, University of Calgary, 2500 University Drive NW, Calgary, Alberta T2N 1N4, Canada ABSTRACT rocks, ranging from Cambrian to Late Triassic The Denali fault, a transcurrent fault system that extends from northwestern Canada age, while the Wrangellia terrane is a late Paleo- across Alaska toward the Bering Sea, is partitioned into segments that exhibit variable zoic island-arc assemblage overlain by Mesozo- levels of historical seismicity. A pair of earthquakes (M 6.2 and 6.3) on 1 May 2017, in ic flood basalts and sedimentary rocks (Cobbett proximity to the Eastern Denali fault (EDF), exhibited source mechanisms and stress con- et al., 2016). During the Jurassic and Cretaceous, ditions inconsistent with expectations for strike-slip fault activation. Precise relocation of the Insular superterrane was intruded by several ∼1500 aftershocks revealed distinct fault strands that are oblique to the EDF. Calculated older arcs, and later by the active Wrangell arc, patterns of Coulomb stress show that the first earthquake likely triggered the second one. which has migrated from southeast to northwest The EDF parallels the Fairweather transform, which separates the obliquely colliding Ya- since 28 Ma (Beranek et al., 2017). kutat microplate from North America. In our model, inboard transfer of stress is deforming The Yakutat microplate is presently mov- and shortening the mountainous region between the EDF and the Fairweather transform. ing northwest relative to the North American This is supported by historical seismicity concentrated southwest of the EDF, suggesting plate and is obliquely colliding with the western that it now represents a structural boundary that controls regional deformation but is no continental margin (Fig. 1). The margin-paral- longer an active fault. lel component is largely accommodated by the Fairweather fault (FF) system, which separates INTRODUCTION with important implications for earthquake the Yakutat microplate from North America (El- The Denali fault (DF) is a 2100-km-long, hazards that could impact Whitehorse, Yukon, liott et al., 2010). As the plate boundary bends dextral strike-slip fault in northwestern Canada and Juneau, Alaska. to the west, dextral strike-slip displacement is and Alaska (Fig. 1). In Alaska, the Central De- To address this issue, this study investigated transferred northward to connect with the ac- nali fault (CDF) has attracted considerable atten- the stress regime and tectonic implications of the tive Totschunda fault and CDF via the Connec- tion, particularly since the M 7.9 Denali earth- St. Elias earthquake sequence. The earthquake tor fault (Fig. 1). Historical seismicity reveals quake in 2002 that resulted in surface rupture doublet and prolonged aftershock sequence diffuse deformation with a mix of reverse and extending >340 km (Fig. 1; Eberhart-Phillips were exceptionally well recorded by EarthScope strike-slip faulting in the region between the FF et al., 2003). This earthquake initiated on a pre- Transportable Array stations. Our results indi- and the St. Elias sequence (Fig. 2A). viously unrecognized fault strand and thereupon cate that the current stress regime does not favor The geomorphic expression and inferred activated the CDF, but it also splayed southward slip along the EDF; instead, deformation is dis- slip rate of the DF diminish to the east (Fig. 1; along the Totschunda fault, thus bypassing the tributed across the Insular superterrane between Haeussler et al., 2017). Although the DF has ac- Eastern Denali fault (EDF). the active Yakutat–North American plate bound- commodated ∼400 km of dextral slip since the On 1 May 2017, two earthquakes of M 6.2 ary and the Yukon-Tanana terrane. Early Cretaceous (Lowey, 1998), i.e., an average and 6.3 occurred during a 2 h interval near the rate of >3 mm/yr, the present-day motion of the border between Yukon and British Columbia, TECTONIC BACKGROUND EDF is <1 mm/yr (Marechal et al., 2018). The Canada, in proximity to the EDF (Fig. 2). Pre- The western margin of North America is EDF is connected to the Queen Charlotte fault vious studies of the focal mechanisms of these composed of oceanic and island-arc terranes by the dextral Chatham Strait fault, which has events, as well as historical seismicity in the that accreted during the Mesozoic Era (Fig. 1). been quiescent since ca. 13 ka (Brothers et al., vicinity, reveal a mix of reverse and strike-slip The DF separates the Insular superterrane from 2018). Nevertheless, it remains unclear if the faulting styles (Doser and Rodriguez, 2011; the pericratonic Yukon-Tanana (YT) terrane, a EDF is active, or if deformation has been fully He et al., 2018; Feng et al., 2019). This se- part of the Intermontane belt. The YT terrane transferred to the DRF or other, as-yet unrecog- quence, herein named the St. Elias earthquake is composed of a mid-Paleozoic volcanic-plu- nized, faults in this remote region. sequence, has been interpreted as reactivation tonic assemblage that was strongly deformed of the Duke River fault, a southwest-dipping, and metamorphosed by Late Triassic time and METHODS terrane-bounding structure that runs subpar- subsequently intruded by the coast plutonic Seismic waveforms for the 2017 earthquake allel to the EDF (He et al., 2018). Interfero- arc (Mortensen, 1992; Israel et al., 2014). The doublet and associated aftershocks were ob- metric synthetic aperture radar (InSAR) and Wrangellia and Alexander terranes are separated tained from >60 stations, three of which were GPS data support the interpreted fault geom- by the Duke River fault (DRF) and together form located <50 km from the sequence (Fig. 2B, in- etry (Feng et al., 2019), but the possibility the Insular superterrane. The Alexander terrane set). Using initial locations reported by the U.S. of future activity on the EDF is unresolved, consists of siliciclastic, carbonate, and volcanic Geological Survey (USGS, 2018), we applied CITATION: Choi, M., Eaton, D.W., and Enkelmann, E., 2021, Is the Eastern Denali fault still active?: Geology, v. 49, p. 662–666, https://doi .org/10.1130/G48461.1 662 www.gsapubs.org | Volume 49 | Number 6 | GEOLOGY | Geological Society of America Downloaded from http://pubs.geoscienceworld.org/gsa/geology/article-pdf/49/6/662/5323365/g48461.1.pdf by guest on 27 September 2021 Figure 1. Tectonic setting and generalized geology of Yakutat indentor-corner region in northwestern Canada and southern Alaska. Green and red polygons and arrows indicate Yakutat micro- plate and plate motion vectors at 6 Ma and 0 Ma, respectively, based on the global plate recon- struction model of Müller et al. (2019). Western, Cen- tral, and Eastern Denali fault segments are indi- cated. Inset shows area covered in figure and dis- tribution of seismograph stations used in this study (black triangles). DRF—Duke River fault; TF—Totschunda fault; Connt. F.—Connector fault. Ovals identify fault slip-rate estimates (mm/ yr) from (1) Matmon et al. (2006); (2) Marechal et al. (2018); and (3) Haeussler et al. (2017). Black plate- motion vectors are from Plattner et al. (2007) and Elliot et al. (2010). a double-difference algorithm (Waldhauser and fault based on the Coulomb failure criterion, the initial mainshock. The non-DC components Ellsworth, 2000) to relocate 1498 events. By in which failure is promoted when the stress may be indicative of coseismic brittle damage significantly improving the precision of rela- change is positive (Toda et al., 2011). Details and/or intersecting, overlapping, or nonplanar tive event locations, this technique aids in elu- of the methods used in this study are provided faults (Zhang et al., 2016), suggesting that new cidating the underlying fault architecture. Hi- in the Supplemental Material1. fractures were created within the source region erarchical clustering was then applied to these (Julian et al., 1998). relocated events to group events on the basis RESULTS The relocated events revealed distinct clouds of waveform similarity. Using this approach, Compared with previous investigations (He of seismicity in a depth range of 4–15 km, il- events were classified into 136 clusters, of et al., 2018; Feng et al., 2019), novel aspects luminating inferred fault planes (Fig. 3). As which the three largest clusters included more of our analysis included stress inversion, depth indicated by interpreted fault segments (bold than 37% of all events. Moment tensors (MTs) dependence of Coulomb stress change, detailed white lines in Fig. 2B), the orientation of the were calculated by full-waveform inversion us- aftershock analysis (clustering), consideration major axis of each aftershock cloud is aligned ing a grid search and generalized least-squares of the regional geological context, and interpre- with one nodal plane of the corresponding fo- method. Using the best-fitting double-couple tation of changes in plate motion and tectonic cal mechanism. Waveform-based hierarchical mechanisms for the seven largest events, ori- history that produced the inferred stress regime. clustering analysis revealed internal details of entations of the principal stress axes and their Our MT inversions confirm that the first main- the sequence as it migrated from the first to associated uncertainties were determined in the shock was characterized by oblique-reverse slip the second fault via a diffuse connecting re- epicentral region using linear stress inversion. on a west-dipping fault (based on aftershocks) gion. A subparallel fault strand west of the first This stress regime is applicable to the depth with a significant non-double-couple (non- mainshock was also activated, accompanied by range of the mechanisms used in the inversion DC) component (∼40%).
Details
-
File Typepdf
-
Upload Time-
-
Content LanguagesEnglish
-
Upload UserAnonymous/Not logged-in
-
File Pages5 Page
-
File Size-