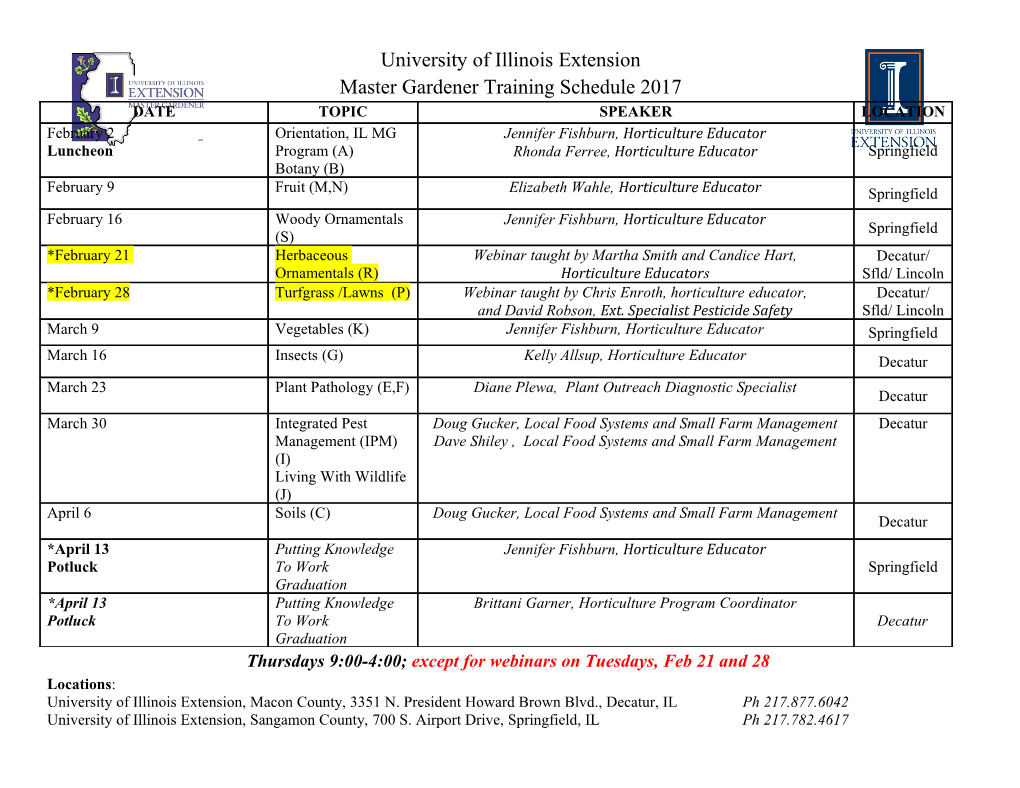
Diss. ETH No. 19606 Microscopic Probing and Manipulation of Ultracold Fermions A dissertation submitted to the ETH ZURICH for the degree of Doctor of Sciences presented by TORBEN MÜLLER Dipl.-Phys., Johannes Gutenberg-Universität Mainz, Germany born 18.06.1980 in Weilburg, Germany citizen of Germany accepted on the recommendation of Prof. Dr. Tilman Esslinger, examiner Prof. Dr. Jonathan Home, co-examiner 2011 Kurzfassung Diese Arbeit beschreibt Experimente, die erstmals einen lokalen Zugang zur mikrosko- pischen Quanten-Vielteilchen-Physik ultrakalter atomarer Fermionen erlauben. Ein Quantengas aus 6Li Atomen wird in-situ mit zuvor nie erreichter Auflösung un- tersucht, wodurch ein direkter Einblick in die charakteristischen Fluktuations- und Korrelationseigenschaften des Systems gegeben ist. Auf derselben Längenskala von einem Mikrometer wird mit flexibel formbaren optischen Dipolfallen zudem eine lo- kale Manipulation erreicht. Wichtigstes Instrument für die gezeigten Experimente bilden zwei identische hochauflösende Mikroskopobjektive, die das Kernstück der im Rahmen dieser Arbeit aufgebauten Apparatur darstellen. Eines der beiden Mikroskopobjektive dient zur hochauflösenden Abbildung. Mit des- sen Hilfe werden räumlich aufgelöste Dichte- und Dichtefluktuationsprofile eines ge- fangenen, schwach wechselwirkenden Fermi-Gases gemessen und analysiert. Für Quan- tenentartung zeigt das Fermi-Gas unterdrückte Dichtefluktuationen unterhalb des Niveaus für thermisches Schrotrauschen. Dahingegen wird im nicht entarteten Fall thermisches, atomares Schrotrauschen beobachtet. Die gemessenen sub-Poisson’schen Fluktuationen sind eine direkte Folge des Pauli-Prinzips und manifestieren damit fer- mionisches Antibunching im Ortsraum. Zudem liefern die ortsaufgelösten Messungen lokale Informationen über thermodynamische Eigenschaften des Systems, wie zum Beispiel den Grad der Quantenentartung und die Kompressibilität. Gestützt auf die Aussagen des Fluktuations-Dissipations-Theorems wird eine neue fluktuationsbasier- te Methode zur Temperaturmessung in atomaren Fermi-Gasen realisiert. Darüber hinaus stellen wir eine neuartige, quanten-limitierte Interferometrie-Methode vor, die es ermöglicht Spinfluktuationen in einem gefangenen, zweikomponentigen Fermi-Gas mit Mikrometerauflösung zu detektieren. Im Vergleich zu einem thermi- schen Gas beobachten wir aufgrund des Pauli Prinzips eine Unterdrückung der Spinf- luktuationen um 4.5dB für ein schwach wechselwirkendes, quantenentartetes Gas. Für ein stark wechselwirkendes Gas aus Feshbach-Molekülen messen wir bedingt durch die Paarbildung von Atomen mit entgegengesetztem Spin eine Reduktion der Fluk- tuationen um 9.2dB. Mit Hilfe eines zweiachsigen akusto-opitschen Deflektors und der neuen hochauflö- senden Optik werden mikroskopisch formbare optische Dipolpotentiale generiert. Die- se umfassen sowohl statische als auch zeitgemittelte Fallenpotentiale in vielfältigen i Geometrien. Wir präsentieren die Charakterisierung einer einzelnen, stark fokussier- ten Dipolfalle und zeigen die Realisierung eines zweidimensionalen optischen Gitters mit 4x4 Gitterplätzen und einer Ringgitterkonfiguration aus 8 Potentialtöpfen. Zu- dem demonstrieren wir das ortsaufgelöste Abbilden von kalten Atomen, die in diesen projezierten optischen Potentiallandschaften eingeladen und gefangen werden. ii Abstract This thesis reports on experiments that provide for the first time a local access to the microscopic quantum many-body physics of ultracold atomic fermions. A quantum gas of 6Li atoms is optically probed in-situ with unprecedented spatial resolution, giving direct insight into the distinctive fluctuation and correlation properties of the system. Likewise on the same length scale of one micrometer, local manipulation is achieved by means of flexible confinement in optical dipole traps. The essential tool for the presented experiments is a pair of identical, high-resolution microscope objectives that constitute the key feature of the new apparatus which has been set up in the scope of this PhD project. Employing one of the two microscope objectives for high-resolution imaging, spa- tially resolved density and density fluctuation profiles of a trapped, weakly interact- ing Fermi gas are measured and analyzed. In the quantum degenerate regime, the Fermi gas shows a suppression of the density fluctuations below the atomic shot noise limit, whereas in the non-degenerate case thermal atomic shot noise is observed. The measured sub-poissonian fluctuations are a direct result of the Pauli exclusion princi- ple and represent an explicit manifestation of antibunching in real space. Moreover, the spatially resolved measurements reveal local information about thermodynamic quantities such as the level of quantum degeneracy and the compressibility. Using the predictions of the fluctuation-dissipation theorem, a novel fluctuation-based method for thermometry in atomic Fermi gases is realized. A novel shot-noise limited interferometer is introduced enabling us to measure the spin fluctuations in a trapped, two-component Fermi gas with a micrometer resolution. Compared to a thermal gas, we observe a reduction of the spin fluctuations of up to 4.5dB for a weakly interacting quantum degenerate gas due to the Pauli principle, and 9.2dB for a strongly interacting gas of Feshbach molecules due to pairing. Using a two-axis acousto-optical deflector in combination with the microscope setup, we demonstrate the generation of microscopically tailored optical dipole potentials. Covering static as well as time-averaged potentials, versatile trapping geometries are achieved, including a tightly focussed single optical dipole trap, a 4x4-site two- dimensional optical lattice and a 8-site ring lattice configuration. Moreover, we present the spatially resolved imaging of cold atoms residing in these optically pro- jected potential patterns. iii Contents 1 Introduction 1 2 Quantum degenerate Fermi gases 7 2.1 Degenerate fermions . 8 2.1.1 Non-interacting trapped Fermi gas . 9 2.2 Interactions in an ultracold quantum gas . 11 2.2.1 Elastic scattering . 11 2.2.2 Feshbach resonances . 12 2.2.3 BEC-BCS crossover . 15 2.3 Quantum statistics . 19 2.3.1 Grand canonical ensemble . 19 2.4 Density fluctuations . 21 2.4.1 Fluctuations in phase space . 21 2.4.2 Fluctuations in real space . 25 2.4.3 Density fluctuations in an ultracold Fermi gas of 6Li . 27 2.5 Fluctuation-dissipation theorem . 29 2.5.1 Fluctuations and susceptibilities . 30 2.5.2 Fluctuations and correlations . 31 3 Experimental setup 35 3.1 General design considerations . 35 3.2 Overview of the experimental setup . 37 3.2.1 Cooling strategy . 37 3.2.2 Vacuum system . 38 3.3 Laser cooling . 43 3.3.1 Laser system . 43 3.3.2 Zeeman slower . 45 3.3.3 Magneto-optical trap . 46 3.4 All-optical evaporative cooling . 48 3.4.1 Resonator trap . 49 3.4.2 Running wave dipole trap . 55 3.4.3 Optical transport . 57 3.5 Magnetic fields . 58 v Contents 3.5.1 Main coils . 59 3.5.2 Auxiliary coils . 60 3.6 Imaging techniques . 61 3.6.1 Absorption imaging . 61 3.6.2 Dispersive probing . 63 3.6.3 Optical setup for standard imaging . 64 3.7 Experimental sequence towards quantum degeneracy . 66 3.7.1 Experimental cycle . 66 3.7.2 Quantum degenerate, non-interacting Fermi gas . 68 3.7.3 Bose-Einstein condensate of molecules . 69 4 Microscope setup 71 4.1 Microscope objectives . 71 4.1.1 Objective design . 72 4.1.2 Objective mounting . 73 4.2 High-resolution imaging . 75 4.2.1 Optical setup . 75 4.2.2 Optical performance . 77 5 Microscopically tailored optical potentials 81 5.1 Generation of optical micro-potentials . 82 5.1.1 Basic concept . 82 5.1.2 Optical setup . 82 5.2 Optical micro-potentials . 84 5.2.1 Single spot micro-trap . 84 5.2.2 Multiple spot micro-traps . 85 5.3 Atoms in micro-potentials . 87 5.3.1 Loading the micro-traps . 87 5.3.2 Single-site resolved imaging . 88 5.3.3 Lifetime in micro-traps . 89 5.4 Summary . 90 6 Local observation of antibunching 91 6.1 Introduction . 92 6.2 Probing density fluctuations in a trapped Fermi gas . 92 6.2.1 Preparation and measurement procedure . 93 6.2.2 Data processing . 94 6.3 Manifestation of antibunching in real space . 96 6.3.1 Density fluctuations above and below quantum degeneracy . 96 6.3.2 Density fluctuation profiles . 98 6.4 Fluctuation-based thermometry . 100 6.5 Summary . 101 7 A local interferometer probing spin fluctuations in a quantum gas 103 7.1 Local interferometry . 103 7.1.1 Concept of the local interferometer . 104 vi Contents 7.1.2 Imprinting atomic spin onto light phase . 105 7.1.3 High-precession at the shot noise limit . 106 7.2 Probing spin fluctuations in a two-component Fermi gas . 107 7.2.1 Spin fluctuations in a weakly interacting Fermi gas . 108 7.2.2 Spin fluctuations in a strongly interacting Fermi gas . 109 7.3 Magnetic susceptibility . 110 7.4 Summary . 111 8 Conclusions and outlook 113 A Physical constants 117 B Atomic properties of 6Li 119 B.1 Fundamental physical properties of 6Li . 119 B.2 Atomic level structure of 6Li . 120 B.3 Zeeman splitting of ground and excited state levels . 121 C Noise propagation 123 D Data processing for interferometry 125 E Alignment of the microscope setup 127 Bibliography 131 Publications related to this thesis 145 Acknowledgements 147 Curriculum Vitae 149 vii 1 Introduction The study of quantum many-body physics has long since captured the interest of physicists, most of which has been sparked by the serendipitous discovery of new states and properties in the field of condensed matter physics. The emergence of phe- nomena such
Details
-
File Typepdf
-
Upload Time-
-
Content LanguagesEnglish
-
Upload UserAnonymous/Not logged-in
-
File Pages160 Page
-
File Size-