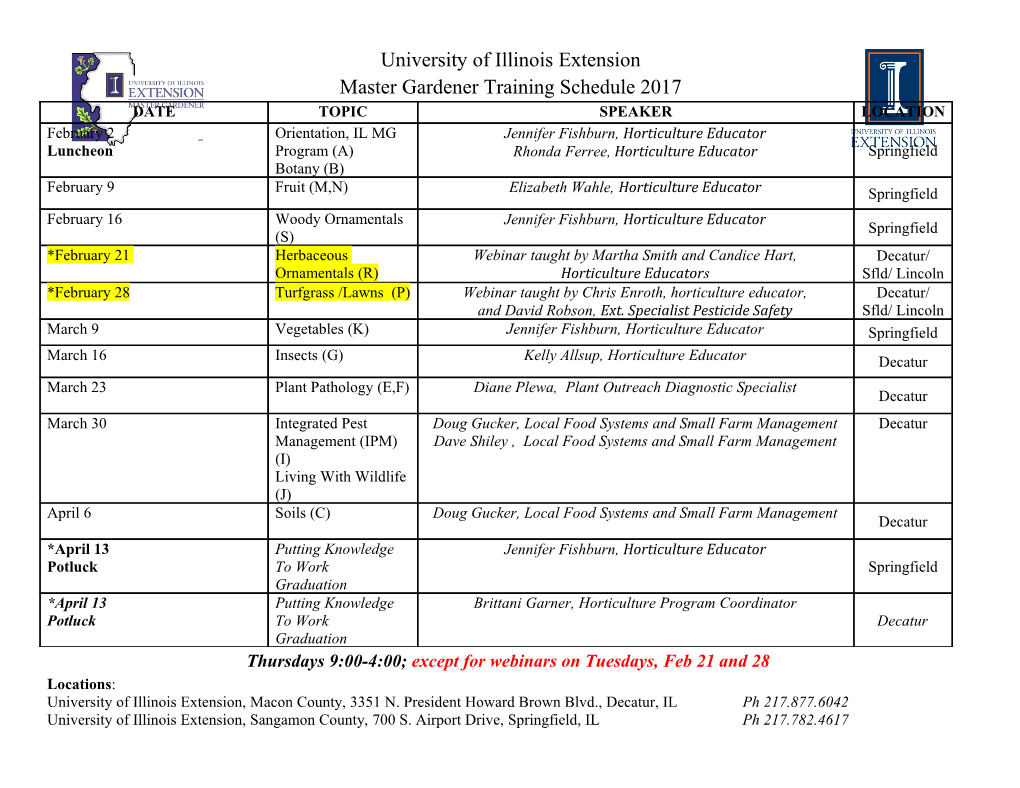
Applications of nanotechnology in electronics pdf Continue Part of a series of articles on Nanoelectronics Single-Molecular Electronics Molecular Scale Molecular Logic Gate Molecular Wires Solid-State Nanocircuitry Nanowires Nanolithography NEMS Nanosensor Moore Law Multigate Device Semiconductor Device Manufacturing List of Examples of Semiconductor Nanoionics Nanophotonics Nanomechanics Science Portal Electronics Portal Technology portal portal portalvte Part of a series of articles on Nanotechnology Stories Organization Popular Culture Outline Impact and Applications Nanomedicine Nanotoxicology Green Nanotechnology Dangers Regulation of Fuller Nanomaterials Carbon Nanoparticles Nanoparticles Molecular Self-Assembly Self-Assembling Supramolecular Assemblage DNA Nanoelectronics Molecular Scale Electronics Nanolithography Moore Law Semiconductor Device Manufacturing Semiconductor Scale The Electron microscope microscope microscope Super resolution microscopy Nanotribology Molecular nanotechnology Molecular Nanorobotics Mechano molecular engineering science portalvte Nanoelectronics refers to the use of nanotechnology in electronic components. The term covers a wide range of devices and materials, with a common characteristic that they are so small that interatomic interactions and quantum-mechanical properties must be studied extensively. Some of these candidates include: hybrid molecular/semiconductor electronics, one-dimensional nanotubes/nanowires (e.g. silicon nanowires or carbon nanotubes) or advanced molecular electronics. Nanoelectronic devices are critical in size with sizes ranging from 1 nm to 100 nm. The latest generations of MOSFET silicon technologies (metal oxide- semiconductor transistor, or MOS transistor) are already under this mode, including 22 CMOS (additional MOS) nodes and the subsequent 14 nm, 10 nm and 7 nm FinFET (fin-field transistor). Nanoelectronics is sometimes seen as a disruptive technology because the current candidates are significantly different from traditional transistors. Fundamental Concepts In 1965, Gordon Moore noticed that silicon transistors were undergoing an ongoing process of scaling down, an observation that was later codified as Moore's Law. Since its observation, the minimum size of transistor objects has decreased from 10 micrometers to 10 nm from 2019. Note that the technology node does not directly represent the minimum size of the object. The field of nanoelectronics aims to ensure the continuation of this law through new methods and materials to create electronic devices with the size of functions at the nanoscale. Mechanical Problems Object Volume Decreases as Third Force linear sizes, but the surface area only decreases his second strength. This somewhat subtle and inevitable principle has enormous implications. For example, the power of the drill (or any other machine) is proportional to the volume, while the friction of the bearings and gear drills is proportional to their surface area. For a normal-sized drill, the power of the device is enough to conveniently overcome any friction. However, scaling its length down 1,000 times, for example, reduces its power by 10,003 (a billion) while reducing friction by only 10,002 (a ratio of only a million). Proportionately it has 1000 times less energy per friction unit than the original drill. If the initial friction-to-power ratio was, say, 1%, it means that a smaller drill would have 10 times more friction than power; drill is useless. For this reason, while super-miniature electronic integrated circuits are fully functional, the same technology cannot be used to make working mechanical devices outside the scales, where frictional forces begin to exceed available power. So even if you can see microphotographs delicately etched silicon gear, such devices are now little more than curiosities with limited real-world applications, such as in moving mirrors and shutters. Surface tension increases in much of the same way, thus increasing the tendency for very small objects to stick together. This could make any kind of micro-factory impractical: even if robotic weapons and hands can be reduced, anything they pick up is usually impossible to put down. The aforementioned, molecular evolution led to the work of cilia, flagella, muscle fibers and rotary motors in aqueous environments, all at the nanoscale. These machines use increased frictional forces found on the micro or nanoscale. Unlike a paddle or propeller, which depends on normal frictional forces (frictional forces perpendicular to the surface) to achieve propulsion, cilia develop movement from exaggerated resistance forces or laminar (frictional forces, parallel surfaces) present in micro and nanoscale. To create meaningful machines at the nanoscale, you need to take into account the appropriate forces. We are faced with the development and design of internally appropriate machines, rather than simple reproductions of macroscopic machines. Therefore, all scaling issues should be carefully evaluated when evaluating nanotechnology for practical applications. Approaches Nanofabrication Main articles: Nanocircuitry and nanolithography For example, electronic transistors that include transistor operation based on a single electron. Nanoelectromechanical systems also fall into this category. Nano-fabrication can be used to build parallel arrays of nanowires, as an alternative to the synthesis of nanowires separately. The silicon nanowires of nanowires have a particular focus in this area increasingly being studied in the direction of various applications in nanoelectronics, energy conversion and storage. Such SiNWs can be manufactured by thermal oxidation in large quantities to produce guided thickness nanowires. Nanomaterials electronics In addition to being small and allowing more transistors to be packed into a single chip, the uniform and symmetrical structure of nanowires and/or nanotube allows for higher electron mobility (faster electron movement in the material), higher dielectric constant (fast frequency), and symmetrical electron/hole characteristic. In addition, nanoparticles can be used as quantum dots. Molecular Electronics Main article: Molecular Electronics Scale Single Molecule Devices is another possibility. These circuits will make heavy use of molecular self-sumen, designing the components of the device to create a larger structure or even a complete system of its own. This can be very useful for reconfigurable computing, and can even completely replace the current FPGA technology. Molecular electronics is a new technology that is still in its infancy, but also offers hope for truly atomic electronic systems in the future. One of the most promising applications of molecular electronics was proposed by IBM researcher Ari Aviram and theoretical chemist Mark Ratner in their 1974 and 1988 works Molecules for Memory, Logic and Amplification (see incredible fixer). This is one of many possible ways to synthesize a molecular-level diode/transistor using organic chemistry. The model system was proposed with a carbon spiro structure, giving a molecular diode about half a nanometer through which polythiophene molecular wires can be connected. Theoretical calculations have shown that the design is in principle audible, and there is still hope that such a system can be earned. Other Nanoionics approaches study the transport of ions rather than electrons in nanoscale systems. Nanophotonics studies the behavior of light on a nanoscash, and aims to develop devices that use this behavior. History See also: History of nanotechnology, semiconductor manufacturing and transistor quantity In 1960, Egyptian engineer Mohamed Atallah and Korean engineer Dovon Kang of Bell Labs manufactured the first MOSFET (semiconductor field transistor) with a 100 nm thick gate oxide, In 1962, Atallah and Kang produced a nano-base metal structure, which used 10 nm thick gold (Au) thin films. In 1987, Iranian engineer Bijan Davari led ibm's research team, which demonstrated the first MOSFET with a 10 nm thick gate oxide using the technology Multi-gate MOSFETs have allowed scaling below 20 nm length gate, starting with FinFET (fin field transistor effect), transistor), non-planetary, double mosFET gates. FinFET comes from the DELTA transistor developed by Dig Hisamoto, Toru Kagoi, Yoshifumi Kawamoto and Eiji Takego in 1989. In 1997, DARPA contracted a research team at the University of California, Berkeley, to develop a deep delta transistor. The group consisted of Hisamoto along with Chenming Hu TSMC and other international researchers including Tsu-Je King Liu, Jeffrey Bokora, Hideki Takeuchi, K. Asano, Yakub Kedzierska, Xuejue Huangga, Leland Chang, Nick Lindert, Shibli Ahmed and Cyrus Taberi. The team successfully manufactured FinFET devices up to 17 nm in 1998 and then 15 nm in 2001. In 2002, the band, including Yu, Chang, Ahmed, Hu, Liu, Bokor and Tabery, manufactured the 10-nm FinFET device. In 1999, the CMOS transistor (Additional MOS), developed at the Electronics and Information Technology Laboratory in Grenoble, France, tested the limits of the 18 nm MOSFET transistor (approximately 70 atoms side by side). This allowed the theoretical integration of seven billion compounds on coin No. 1. However, the CMOS transistor was not an easy research experiment to study how CMOS technology works, but rather a demonstration of how this technology functions now that we ourselves are getting closer to working on a molecular scale. According to the words in 2007, it would be impossible to master the
Details
-
File Typepdf
-
Upload Time-
-
Content LanguagesEnglish
-
Upload UserAnonymous/Not logged-in
-
File Pages3 Page
-
File Size-