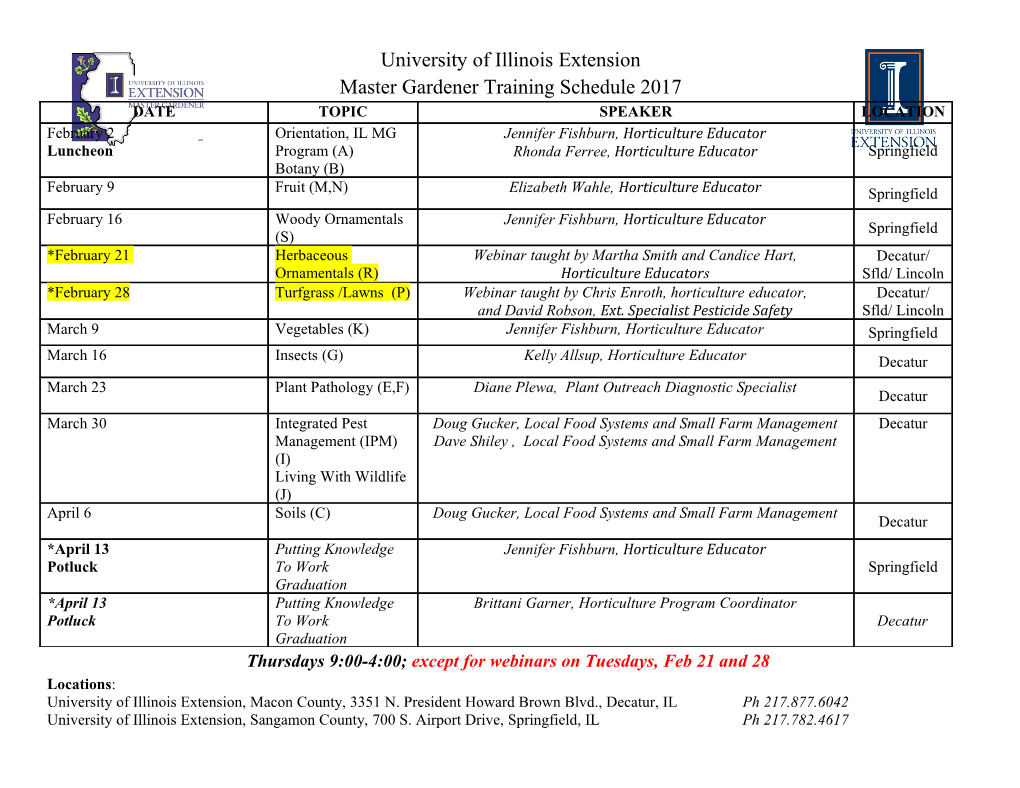
Laser-driven discharges and electromagnetic fields Philip Wykeham Bradford Doctor of Philosophy University of York Physics December 2020 0.1 Abstract When high intensity lasers interact with solid targets, hot electrons are produced that can exit the material and leave behind a positive electric charge. As this accumulated charge is neutralised by a cold return current, radiation is emitted with characteristics dependent on laser and target properties. This thesis examines how electromagnetic radiation is emitted in experiments with long-pulse and short-pulse lasers. Radiofrequency electromagnetic pulses emitted during ps-duration laser inter- actions can disrupt scientific measurements and damage electronic equipment close to the target. A study of electromagnetic pulses produced by the Vulcan laser is presented. Strong fields exceeding 100 kV/m and 0:1 mT were measured 1:5 m from the target using conducting probes. Scaling of the EMP field with laser and target parameters shows qualitative agreement with target charging models. A novel EMP mitigation scheme is presented using a dielectric spiral target holder. Experimental results are used to benchmark a frequency-domain dipole antenna model of EMP emission that connects charging physics to EMP fields measured at an arbitrary distance from the target. In a separate experiment, coil targets were driven with three ns laser beams from the Vulcan laser, generating multi-tesla quasi-static magnetic fields. Dual- axis proton deflectometry was used to measure electric and magnetic fields around the coils. Results suggest that wire electric fields of order 0:1 GV/m develop on a 100 ps timescale. Maximum currents of 10 kA were observed towards the end of the laser drive for 1 mm- and 2 mm-diameter loop targets, corresponding to an axial magnetic field of B0 ≈ 12 T in the 1 mm loops. Deflectometry results agree well with a plasma diode model, whereas B-dot probe measurements of the magnetic field were approximately 10× larger. Analytic and computational modelling of charged particle motion in electric and magnetic fields is presented. Prospects for an all-optical platform for magnetized high energy density physics experiments are discussed. 2 Contents 0.1 Abstract . .2 0.2 Acknowledgements . 23 0.3 Declaration . 25 I Introduction 27 1 Plasmas, ICF and EM fields 28 1.1 The Plasma State . 29 1.1.1 Definition of a Plasma . 29 1.1.2 Debye Shielding . 31 1.1.3 Plasma Parameter and Plasma Frequency . 33 1.2 Inertial Confinement Fusion . 34 1.2.1 Magnetized laser-driven ICF . 36 1.3 Pulsed Power Devices . 38 1.4 Capacitor Coils . 39 1.4.1 CO2 Laser Experiment by Daido et al. ............. 41 1.4.2 Multi-probe experiment by Santos et al. ............ 43 1.4.3 Summary of Capacitor Coil experiments . 46 1.5 Laser-driven Electromagnetic Pulses . 48 1.5.1 Introduction to EMP . 48 1.5.2 Vulcan Petawatt experiment by Mead et al. .......... 49 1.5.3 Lawrence Livermore EMP Campaign . 51 1.5.4 EMP Campaign at CELIA . 53 1.6 Research Goals and Thesis Structure . 58 II Laser-plasma Interactions and Target Charging 60 2 High power laser interactions with solid matter 61 2.0.1 Optical Field Ionisation Processes . 61 2.0.2 Single particle motion in a laser field . 62 2.0.3 Laser propagation in uniform plasma . 64 3 CONTENTS 2.0.4 Laser propagation into expanding plasma . 64 2.1 Laser Absorption Mechanisms . 66 2.1.1 Inverse Bremsstrahlung . 66 2.1.2 Resonance Absorption . 67 2.1.3 Ponderomotive Acceleration . 68 2.1.4 Parametric Instabilities . 69 2.1.5 Hot electron temperature . 69 2.2 Hot electron transport and return currents . 71 2.2.1 The Alfv´enLimit . 72 2.3 Plasma Expansion into Vacuum . 75 2.3.1 Planar Isothermal Rarefaction . 76 2.3.2 Planar Isothermal Plasma Expansion . 77 2.4 Target Normal Sheath Acceleration . 83 2.5 Laser-Induced Target Charging . 85 2.5.1 Capacitor model of target charging . 88 2.5.2 ChoCoLaT target charging model . 90 2.6 Capacitor Coil Modelling . 98 2.6.1 Fiksel capacitor coil model . 98 2.6.2 Plasma diode model of a capacitor coil . 106 3 Measuring electromagnetic fields 117 3.1 Conducting probes . 117 3.1.1 B-dot magnetic probe . 118 3.1.2 D-dot electric probe . 119 3.2 Frequency-dependent attenuation correction in coaxial cables . 119 3.3 Proton Deflectometry . 122 3.4 Charged particle motion in uniform EM fields . 125 3.4.1 Ion deflection in a uniform electrostatic field . 126 3.4.2 Ion deflection in a uniform magnetostatic field . 128 3.4.3 Analytic proton deflection in capacitor coil magnetic fields . 131 3.4.4 Analytic proton deflection in capacitor coil electric and mag- netic fields . 137 III Experiments and Analysis 143 4 Laser-Driven Radiofrequency Electromagnetic Pulses 144 4.1 Outline of Vulcan 2017 experiment . 144 4.1.1 Experiment Set-Up . 145 4.2 Experimental Results . 145 4 CONTENTS 4.2.1 EMP variation with laser parameters . 149 4.2.2 EMP variation with target parameters . 153 4.2.3 PIC and EM wave simulations . 155 4.3 Summary of Vulcan EMP experiment . 156 4.4 Frequency-domain dipole model of EMP . 156 4.4.1 Antenna equation for EMP . 160 4.4.2 The target charging time . 161 4.4.3 Discussion of the dipole antenna model . 162 4.4.4 Comparison of dipole model with Vulcan data . 164 4.5 EMP Chapter Summary . 165 5 Laser-driven solenoids: Capacitor Coil targets 166 5.1 Outline of Vulcan Experiment . 166 5.2 Experimental Set-up . 167 5.3 Proton Radiography . 170 5.3.1 Analytic method for extracting the magnetic field . 171 5.3.2 Proton radiography simulations with EPOCH . 173 5.3.3 Perpendicular deflectometry: B-field only simulations . 178 5.3.4 Perpendicular deflectometry: Combined E and B-field simu- lations . 183 5.3.5 Axial deflectometry: Combined E and B-field simulations . 186 5.3.6 Axial deflectometry: Upper limits on capacitor coil magnetic field . 187 5.3.7 Simultaneous dual-axis proton probing . 188 5.3.8 Scaling of proton deflection with proton energy . 191 5.4 Discussion . 192 5.5 B-dot probe results . 194 5.6 Summary of Vulcan capacitor coil experiment . 199 6 Conclusion 201 A Appendix 206 A.1 Equivalent circuits . 206 A.1.1 RC series circuits . 206 A.1.2 RL series circuits . 209 A.1.3 RLC series circuits . 212 5 List of Tables 1.1 Coil current (J) and central magnetic field (B0) measured in exper- iments with capacitor coil targets. Standard capacitor coil targets with a single wire loop connecting two metal plates are denoted by `CC'. All experiments used nanosecond-duration laser pulses to drive the capacitor coil and results are placed in order of increasing Iλ2 [184]. 47 2.1 List of parametric instabilities and their wave products. Note that the wave frequencies are consistent with Eq. (2.13). 69 5.1 Coil current and central magnetic field for two capacitor coil data shots - one with a 1 mm-diameter loop and the other with a 2 mm- diameter loop. Errors in proton energy and probe time are estimated the FWHM of the RCF response function (see Fig. 5.4a), although probe time errors are limited to ±50 ps by experimental factors. Er- rors in the current and magnetic field are calculated from uncertainty in the capacitor coil to RCF distance, D = 70 ± 5 mm, added in quadrature with a representative 1 kA error from the EPOCH simu- lations. 180 5.2 Ratio between the magnetic field at the loop centre to the magnetic field at the probe position, B0=Bprobe, simulated using a Python finite difference code. Values are given to two significant figures. 197 6 List of Figures 1.1 Two electrodes suspended in a plasma will attract a thin layer of charge that screens the electrode voltage from the surrounding material. 31 1.2 In magnetized ICF, a seed magnetic field is applied along one axis of a standard fusion capsule. Assuming ideal MHD, the magnetic field is trapped when the capsule is ionized and the magnetic flux density increases as the capsule is compressed. 37 1.3 Capacitor coil target driven by an energetic ns-duration laser pulse. Typical values of the coil radius R, current I and magnetic field B are included. 40 1.4 (a) Schematic diagram of a wire coil target and the observation sys- tem. (b) X-ray signal (white regions) in the gap between the capacitor coil plates. The laser enters from the left, passes through a hole in the front disk and irradiates the rear disk. Both figures are taken from Daido et al. [46] with permission. 42 1.5 (a) Maximum coil magnetic field and coil current as a function of plate separation. The solid circles and the triangle represent data for a one-turn coil made of wire and a cylindrical target respectively. A schematic of the cylindrical target is shown in the top left hand corner. (b) Maximum plate voltage as a function of plate separation (inferred from current measurements using a lumped-element circuit model). Notice that the voltage is similar for wire and cylinder tar- gets. Figures are taken from Daido et al. [46] with permission. 43 1.6 (a) Photographs of Ni and Cu capacitor coil targets used in the exper- iment (b) Full experimental set-up showing the B-dot probe, Faraday rotation probe beam and proton radiography diagnostics. Figures are taken from Santos et al. [153] with permission. 44 7 LIST OF FIGURES 1.7 (a) Sample RCF image taken using 13 ± 1 MeV protons that crossed the target t ≈ 0:35 ns after the beginning of the laser drive.
Details
-
File Typepdf
-
Upload Time-
-
Content LanguagesEnglish
-
Upload UserAnonymous/Not logged-in
-
File Pages233 Page
-
File Size-