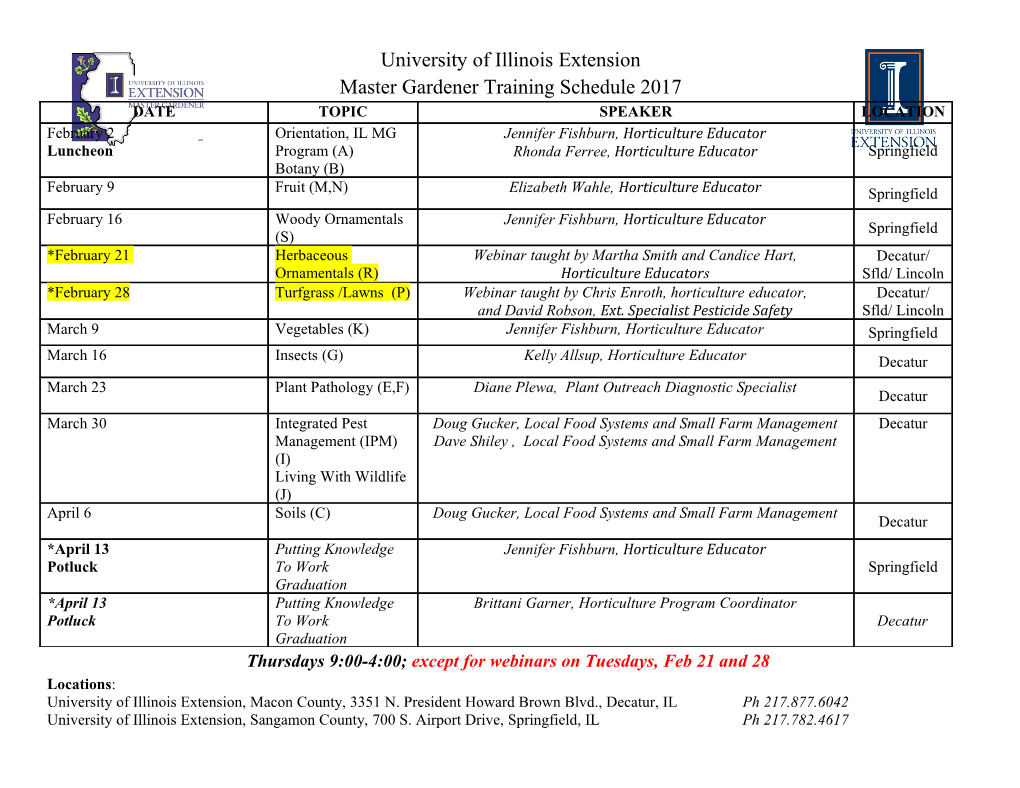
T H E U N I V E R S I T Y of T E X A S S C H O O L O F H E A L T H I N F O R M A T I O N S C I E N C E S A T H O U S T O N Circular Dichroism For students of HI 6001-100 “Computational Structural Biology” Willy Wriggers, Ph.D. Adopted from material by Mathew Baker, Univ. of Bath http://staff.bath.ac.uk/bssmdb/ http://biomachina.org/courses/structures/08.html What is Circular Dichroism? • Circular Dichroism (CD) is a type of absorption spectroscopy that can provide information on the structures of many types of biological macromolecules • It measures the difference between the absorption of left and right handed circularly-polarized light by proteins. CD is used for; • Protein structure determination. • Induced structural changes, i.e. pH, heat & solvent. • Protein folding/unfolding. • Ligand binding • Structural aspects of nucleic acids, polysaccharides, peptides, hormones & other small molecules. Plane Polarized Light E M Direction of propagation E vectors Polarizer Direction of propagation Plane & Circularly Polarized Light •A light source usually consists of a collection of randomly orientated emitters, the emitted light is a collection of waves with all possible orientations of the E vectors. •Plane polarized light is is obtained by passing light through a polarizer that transmits light with only a single plane of polarization. i.e. it passes only those components of the E vector that are parallel to the axis of the polarizer. •Circular polarized light; The E vectors of two electromagnetic waves are ¼ wavelength out of phase & are perpendicular. The vector that is the sum of the E vectors of the two components rotates so that its tip follows a helical path (dotted line). Circularly Polarized Light • Linearly polarized light: Electric vector direction constant - magnitude varies. • Circularly polarized light: Electric vector direction varies - magnitude constant Circularly Polarized Light • Circular polarized light: Electric vector direction varies - magnitude constant • So its in two forms: left and right handed E E Right- Left- handed handed Circular Dichroism • CD measures the difference between the absorption of left and right handed circularly-polarized light. polarized light: • This is measured as a function of wavelength, & the difference is always very small (<<1/10000 of total). After passing through the sample, the L & R beams have different amplitudes & the combination of the two unequal beams gives elliptically polarized light.Hence, CD measures the ellipicity of the transmitted light (the light that remains that is not absorbed): Absorption Spectroscopy • Shine light through a sample and measure the proportion absorbed as a function of wavelength. • Absorbance A = log(I /I) 0 l • Beer-Lambert law: wavelength A(λ) = ε(λ)lc λ ε: extinction coefficient Sample I0 conc. I C • The longer the path or the more concentrated the sample, the higher the absorbance Absorption Spectroscopy • CD measures the difference between the absorption of left and right handed circularly-polarized light: ∆A(λ) = AR(λ)-AL(λ) = [εR (λ) - εL (λ)]lc or ∆A(λ) = ∆ε (λ)lc ∆ε is the difference in the extinction coefficients typically < 10 M-1cm-1 typical ε around 20 000 M-1cm-1 So the CD signal is a very small difference between two large originals. Absorption Spectroscopy • CD is only observed at wavelengths where absorption occurs, in absorption bands. • CD arises because of the interaction between different transition dipoles doing the absorption. As this depends on the relative orientation of different groups in space, the signal is very sensitive to conformation. • So in general ∆ε is much more conformation dependent that ε. • We will concentrate on the “electronic CD” of peptides and proteins below 240nm. This region is dominated by the absorption of peptide bond and is sensitive to changes in secondary structure. • Can also do CD in near UV (look at trp side chains), visible (cofactors etc.) and IR regions. •The peptide bond is inherently asymmetric & is always optically active. • Any optical activity from side-chain chromophores is induced & results from interactions with asymmetrical neighbouring groups. CD Signal is a Small Difference Between Two Large Originals • CD of E.Coli DNA Native ------ Denatured • For instance at 260 nm ∆ε = ~3 M-1cm-1 ε = ~6000 M-1cm-1 • i.e. CD signal 0.05% of original • need to measure signals ~1/100 of this! CD Signals for Different Secondary Structures 80000 -1 EL –ER > 0 60000 α-helix dmol β-sheet 2 40000 random coil 20000 0 EL –ER < 0 -20000 -40000 190 200 210 220 230 240 250 Mean residue ellipicity deg cm in wavelength in nm • These are Fasman standard curves for polylysine in different environments (data from ftp://jgiqc.llnl.gov) CD Spectra of Protein 2ndary Structures -ve band (nm) +ve band (nm) α-helix 222 192 208 β-sheet 216 195 β-turn 220-230 (weak) 205 180-190 (strong) L.H polypro II helix 190 210-230 weak Random coil 200 CD Signals are Sensitive to Secondary Structure CD signals for GCN4-p1 • GCN4-p1 is a coiled–coil: O'Shea et al. Science (1989) 243:538 figure 3: 34µM GCN4-p1 in 0.15M NaCl, 10mM phosphate pH 7.0 80 70 60 0 OC -1 50 50 OC O 40 75 C dmol 2 30 20 10 0 in 1000 cm 1000 deg in θ] -10 [ -20 • 100% helical at 0oC -30 -40 190 200 210 220 230 240 250 260 • It melts to a random coil at wavelength in nm high temperature This is the CD spectrum for an α-helix Applications of CD in Structural Biology •Determination of secondary structure of proteins that cannot be crystallised •Investigation of the effect of e.g. drug binding on protein secondary structure •Dynamic processes, e.g. protein folding •Studies of the effects of environment on protein structure •Secondary structure and super-secondary structure of membrane proteins •Study of ligand-induced conformational changes •Carbohydrate conformation •Investigations of protein-protein and protein-nucleic acid interactions •Fold recognition Why use CD? • Simple and quick experiments • No extensive preparation • Measurements on solution phase • Relatively low concentrations/amounts of sample • Microsecond time resolution • Any size of macromolecule Total Signal for a Protein Depends on its Secondary Structure —— chymotrypsin (~all β) —— lysozyme (mixed α & β) —— triosephosphate isomerase (mostly α some β) —— myoglobin (all α) • Notice the progressive change in θ222 as the amount of helix increases from chymotrypsin to myoglobin Finding Proportion of 2ndary Structures • Fit the unknown curve θu to a combination of standard curves. • In the simplest case use the Fasman standards θt = xαθα + xβθβ + xcθc 80000 •Vary x x and x -1 dmol 60000 α, β c 2 α-helix β-sheet to give the best fit of θ to θ 40000 random coil t u 20000 while xα+ xβ + xc = 1.0 0 -20000 -40000 Mean residue ellipicity in deg cm in deg ellipicity residue Mean 190 200 210 220 230 240 250 wavelength in nm • Do this by least squares minimization (http://www-structure.llnl.gov/Xray/comp/lsq.htm) Example Fit: Myoglobin • In this case: θt = xαθα + xβθβ + xcθc • fits best with xα = 80%, xβ= 0% xc = 20% 80000 -1 dmol 60000 2 α-helix β-sheet 40000 random coil 20000 0 • agrees well with structure -20000 -40000 Mean residue ellipicity in deg cm in deg ellipicity residue Mean 78% helix, 22% coil 190 200 210 220 230 240 250 wavelength in nm For further details: www-structure.llnl.gov/cd/cdtutorial.htm Example Fit (2): GCN4-p1 CD signals for GCN4-p1 O'Shea et al. Science (1989) 243:538 figure 3: 34µM GCN4-p1 in 0.15M NaCl, 10mM phosphate pH 7.0 o 80 •At 0C 100% helix 70 60 0 OC -1 O o 50 50 C O 75 C 0% helix 40 75 C dmol 2 30 20 o 10 • Q: what about 50 C? 0 in 1000 deg cm in θ] θ] -10 [ -20 -30 θ = x θ + x θ -40 190 200 210 220 230 240 250 260 t o o 75 75 wavelength in nm • fits best with fit to GCN4-p1 50OC data 10 xo = 50%, original data 5 best fit with mix of 0OC and 75OC spectra x = 50% -1 75 0 mol 2 • Shows that at 50oC -5 -10 1/2 of peptide α-helix dimer -15 MRE in 1000 degs cm 1/2 of peptide random coil -20 monomer -25 200 210 220 230 240 250 wavelength in nm However, CD Signal Depends Somewhat on Environment • Can see this by looking • But on a coiled-coil breaks down at the effect of trifluoroethanol helical dimer to single helices (TFE) on a coiled-coil similar to Effect of 50% TFE on a coiled-coil 0 GCN4-p1 TM-36 aqueous -5 TM-36 + TFE • TFE induces helicity in all -10 peptides -15 Effect of 50% TFE on a monomeric peptide MRE -20 0 peptide in water peptide in 50% TFE -25 -5 -30 -10 TFE -35 -15 200 210 220 230 240 MRE wavelength in nm -20 TFE -25 • Although 2ndry structure same -30 CD changes -35 200 210 220 230 240 wavelength in nm Lau, Taneja and Hodges (1984) J.Biol.Chem. 259:13253-13261 Best Fitting Procedures Use Many Different Proteins For Standard Spectra • There are many different algorithms. • All rely on using up to 20 CD spectra of proteins of known structure. • By mixing these together a fit spectra is obtained for an unknown. • For full details see Dichroweb: the online CD analysis tool www.cryst.bbk.ac.uk/cdweb/html/ • Can generally get accuracies of 0.97 for helices, 0.75 for beta sheet, 0.50 for turns, and 0.89 for other structure types (Manavalan & Johnson, 1987, Anal.
Details
-
File Typepdf
-
Upload Time-
-
Content LanguagesEnglish
-
Upload UserAnonymous/Not logged-in
-
File Pages39 Page
-
File Size-