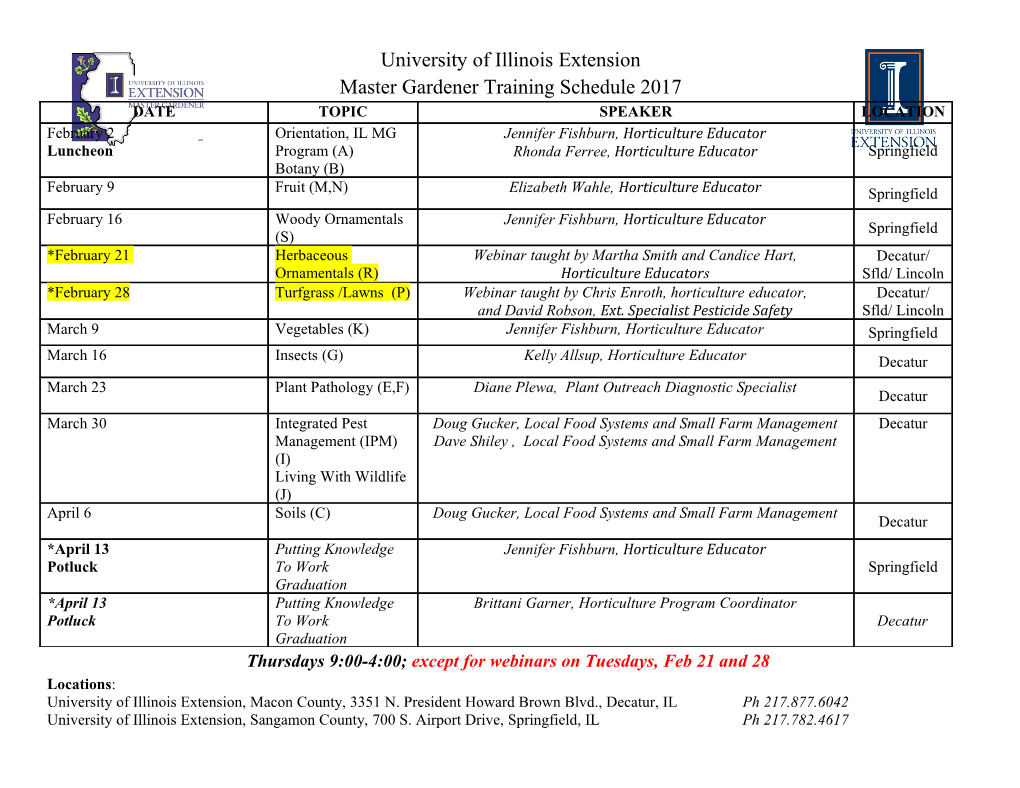
Chapter 4 Living with Pigments: The Colour Palette of Antarctic Life Juan José Marizcurrena, María Fernanda Cerdá, Diego Alem, and Susana Castro-Sowinski Abstract The production of pigments is a common feature that may help microor- ganisms to cope with the harsh conditions found in Antarctica. They have functions such as protection against UV irradiation and superoxide and nitrogen reactive spe- cies (antioxidant activity) and modulation of membrane fluidity under cold stress. In addition, they act as antibiotics, modulating the microbial communities in their natural environments, and harvest light for increasing the efficiency of photosynthe- sis, thus influencing the biogeochemical cycles. This chapter deals with the chemis- try and the biological role of microbial pigments (except chlorophylls) in the Antarctic environment and also includes a brief overview of the potential biotech- nological use of pigments. Keywords Microbial production of pigments · UV-resistance · Antioxidant activity · Membrane fluidity · Photosynthesis · Antimicrobial activity J. J. Marizcurrena · S. Castro-Sowinski (*) Biochemistry and Molecular Biology, Faculty of Sciences, Universidad de la República, Montevideo, Uruguay e-mail: [email protected] M. F. Cerdá Laboratory of Biomaterials, Faculty of Sciences, Universidad de la República, Montevideo, Uruguay D. Alem Biochemistry and Molecular Biology, Faculty of Sciences, Universidad de la República, Montevideo, Uruguay Epigenetics and Genomics Instability Laboratory, Instituto de Investigaciones Biológicas Clemente Estable, Montevideo, Uruguay © Springer Nature Switzerland AG 2019 65 S. Castro-Sowinski (ed.), The Ecological Role of Micro-organisms in the Antarctic Environment, Springer Polar Sciences, https://doi.org/10.1007/978-3-030-02786-5_4 66 J. J. Marizcurrena et al. 4.1 Introduction The ultraviolet (UV) solar radiation is divided into UVC (170–280 nm), UVB (280– 315 nm) and UVA (315–400 nm). UVC, as well as most UVB radiation, does not reach the Earth’s surface due to the filtering effect of the ozone layer located at the stratosphere. This UV irradiation is damaging for the life on Earth, but many life forms produce pigments as protecting molecules among other adaptations to protect themselves. Biological pigments are substances whose presence in tissues or cells colours them. They are found in different sources, as animals and higher plants, but they are also produced by bacteria, algae, lichen or moss. Dyes act as light-harvesting­ molecules, protecting organism from radiation, and are also used for synthetic purposes. During the early stage of life, the selective pressure of solar irradiation may have slanted the microbial evolution (Garcia-Pichel 1998; Cockell and Horneck 2001). The new life forms should have to develop different mechanisms to cope with the harmful effect of solar irradiation, and probably, by natural selection, they produced different pigments as cellular products that served for multiple purposes. Pigments conferred unique features, such as photon harvesting for energy income, and protec- tion of vital molecules like DNA and proteins (Wynn-Williams et al. 2002).Thus, constant UV irradiation would have been shielded by these new pigments, avoiding oxidative stress and direct DNA damage, protecting cells from the UV mutagenic potential (Mulkidjanian and Junge 1997). Currently, as a consequence of the depletion of the ozone layer, organisms from Earth are exposed to increased levels of UV irradiation. Therefore, various organ- isms have developed mechanisms for protection against the damage caused by UV irradiation. They can produce and accumulate absorbing molecules to decrease the transmission of incident light. Alternatively, they can produce UV screening com- pounds such as phenolic compounds, able to absorb and re-emit the UV radiation as fluorescence or thermal radiation. A molecule is able to absorb light from the HOMO to the LUMO orbital, and then several pathways can dissipate the energy. The energy can return to the basal state dissipating the excess of energy by produc- ing heat or by radioactive processes (fluorescence or phosphorescence). Instead, transfer to other molecules, inducing structural modifications via reversible or irre- versible pathways, can dissipate the energy. Finally, the last protective strategy is the production of repairing macromolecules such as the production of photolyases, enzymes that repair the DNA damage induced by UV irradiation (Sancar et al. 2004). Among others, Antarctic bacteria are a good source of highly efficient pho- tolyases (Marizcurrena et al. 2017). This chapter deals with the chemistry and the biological role of pigments pro- duced by microorganisms, with the focus in Antarctic microbes. We will describe a set of pigments, but the well-known photosynthetic pigment chlorophyll has not been included in this chapter. 4 Living with Pigments: The Colour Palette of Antarctic Life 67 4.2 Living with Carotenoids Carotenoids are organic liposoluble pigments from the group of isoprenoids, found naturally in plants and other photosynthetic organisms, and are responsible for most of the yellow, orange or red colours in nature. They are divided into two groups: (1) non-oxygenated molecules as β-carotene and (2) oxygenated molecules as xantho- phylls (Fig. 4.1). The maximum absorptions of carotenoids are between 440 and 520 nm, with a strong molar absorption coefficient (ca. 105 L mol−1 cm−1). They protect cells from photo-oxidative damage by (i) preventing the formation of reac- tive oxygen species through the thermal dissipation of the excess of energy; and (ii) quenching of the excited states of chlorophyll and singlet oxygen. During their emergence in the early life, carotenoids may have function as light-­harvesting antenna, acting as accessory pigments for chlorophylls. As carot- enoids collect energy from a wider spectrum of visible light compared with chlo- rophylls, it may increase the photosynthetic efficiency (Lichtenthaler 1987), thus a OH b HO O c O O HO d O O O OH e HO Fig. 4.1 Structures of (a) β-carotene (b) and lutein (c) cathaxanthin (d) fucoxanthin and (e) zeaxanthin 68 J. J. Marizcurrena et al. influencing the biogeochemical cycling of carbon. On a geological time scales, the evidence support that there is an important impact of photosynthesis in bio- geochemical cycles (Falkowski 1994). Pigments also provide photoprotection against UV irradiation, absorbing light and preserving DNA and proteins from photodamage and oxidizing agents that produce reactive oxygen species (ROS; antioxidant properties). Alternatively, carotenoids provide protection against other physiological stresses such as low temperature, another common feature in Antarctica (Tian and Hua 2010). Carotenoids are also involved in cell differentiation and cell cycle regulation. They also act as growth factor regulators, as immune systems inducers and as intra- cellular signalling molecules, among others functions (Fiedor and Burda 2014). Chemically, they have hydrophobic chains that attach them into the lipid bilayer of cell membranes; thus, changes in the amount of membrane carotenoids influence the thickness, fluidity and rigidity of the membranes, an important feature in a roast- ing early habitat and also in the cold Antarctic environment (Wisniewska and Subczynski 1998, 2006). The advantage of counting on a wide range of carotenoids (β-carotenes and xanthophylls) not only influences the stability of the cell mem- brane, it also improves the resistance to ROS. Carotenoids like β-carotene absorb UVA and UVC light, both inducing oxidative stress and DNA photodamage, respectively. In Antarctica, microorganisms have been detected in all habitats such as lakes, ponds, rivers, streams, rocks and soil. Among pigments, carotenoids are the main pigments found in microbes. They have been reported as cryo- or solar radiation protectants as well as light harvesters in photovoltaic cells (Montagni et al. 2018). Carotenoids such as nostoxanthin and zeaxanthin have been found in Pseudomonas, whereas canthaxanthin or 2′-hydroxyflexixanthin has been found in Hymenobacter isolates (Klassen and Foght 2008). Also the phytoplankton is a rich source of pig- ments including chlorophylls, xanthins and α- and β-carotenes; these photosynthetic and photoprotective pigments may allow the adaptation of life under low light con- ditions (Ferreira et al. 2017). Among other carotenoid-producing Antarctic microorganisms, it has been shown that the bacterium Sphingobacterium antarcticus produces at least three carotenoids (zeaxanthin, β-cryptoxanthin and β-carotene). Another example of an Antarctic carotenoid-producing bacterium is Arthrobacter agilis, isolated from a sea ice sam- ple, that increases the content of carotenoids (C-50 bacterioruberin-type carotenoid and its glycosylated derivatives) at low temperatures of growth; interestingly, the production of carotenoid decreased when growing at high-salinity conditions (Fong et al. 2001). In addition to cold adaptation, the production of carotenoids by Antarctic heterotrophic bacteria is a strategy to withstand other environmental stresses, such as freeze-thaw cycles and solar irradiation (Dieser et al. 2010). The production of carotenoid-like pigments by Arctic microorganisms is also involved in physiological plasticity, a rapid response to a gradual decrease in temperature and freeze-thaw (Singh et al. 2017). Finally, the very well-known radio-resistant bacte- rium Deinococcus radiodurans produces the carotenoid deinoxanthin,
Details
-
File Typepdf
-
Upload Time-
-
Content LanguagesEnglish
-
Upload UserAnonymous/Not logged-in
-
File Pages18 Page
-
File Size-