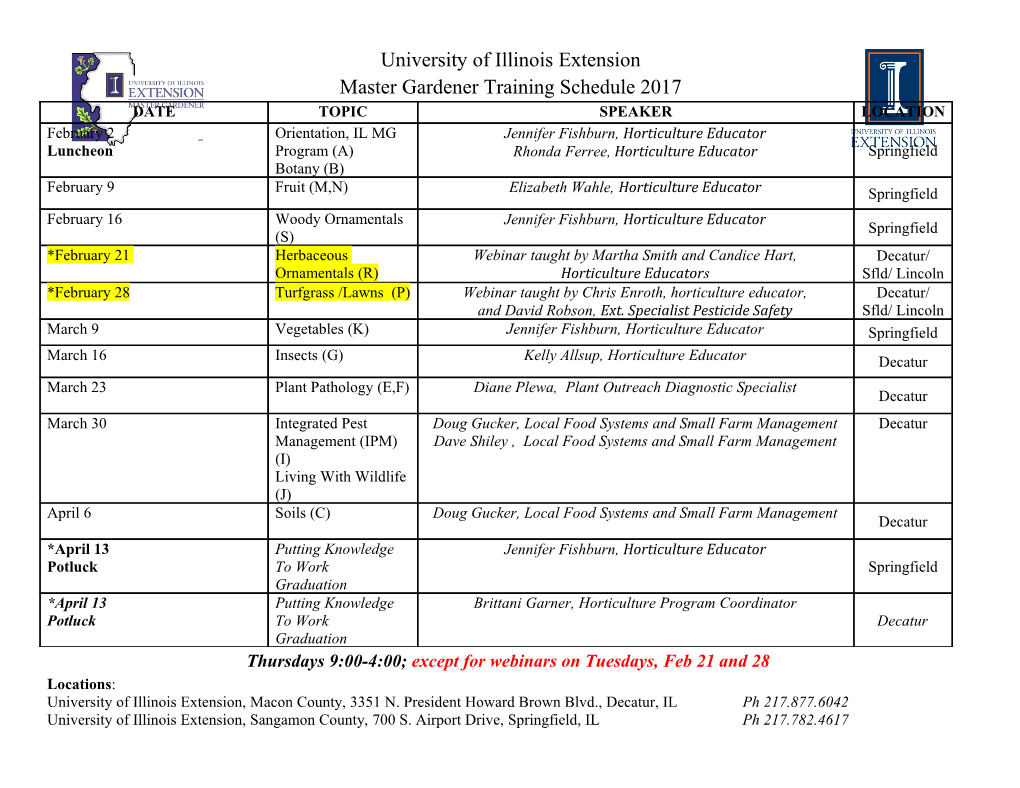
PROGRESS REPORT on CONTROLLED FUSION RESEARCH* by M. H. BRENNAN October, 1972 School of Physical Sciences The Flinders University of South Australia Bedford Park, South Australia 5042 'rReport prepared for the Australian Atomic Energy Commission. 1. INTRODUCTION In this report a survey is made of the international research programme directed towards the development of a controlled thermonuclear fusion reactor. The report is based on observations made during an overseas trip which included attendance at a meeting of the international Fusion Research Council and the Fifth European Conference on Controlled Fusion and Plasma Physics. The itinerary for the trip, which was from 13th August to 28th September, 1972, is included as Appendix A to this report. In order to provide a basis for evaluating the fusion research programme a brief summary is given in Section 2 of the basic requirements for a fusion reactor. In Sections 3 and k of the report brief summeries are given of the main features of the Council meeting and the Fifth European Conference. The current state of fusion research is reviewed in Section 5° The final Section of the report includes a discussion of the present and near-future international fusion research programme, and some comments on the Australian programme. 2. THE FUSION REACTOR 2.1 The Scientific Problem The most favourable nuclear reaction for the release of nuclear energy from the fusion of light elements is the deuterium-tritium reaction (D-T reaction). This reaction has a cross section, at energies of -100 keV, of several hundred times that for the D-D reaction (deuterium-deuterium). In addition, considerably more energy is released per interaction. We therefore concentrate our attention on the D-T reaction; it may be possible, at a later stage, to develop a reactor based on the D-D reaction. The reaction involved is 1H2 + 1H3 -*- aHe" + n1 + (17.6 Mev) . Approximately 14 Mev of the released energy is carried away by the neutron. The deuterium fuel is, of course, available from sea water; the total energy available is sufficient to satisfy the world's electrical energy requirements - 2 - for -JO years. The tritium fuel is, of course, not available naturally and is therefore produced in the reactor system, using a lithium blanket, which also serves as a neutron moderator and is a component of the heat exchange system. In order to achieve the high particle energies required, the fuel (a 50/50 mixture of deuterium and tritium) must be heated to a temperature of ~2 x 108 K, corresponding to a value of kT of 20 keVc At these high temperatures, the atoms of the fuel are all ionized and the gas is in the plasma state. In addition to the temperature requirement, the plasma particles must be contained in the reaction region for a sufficiently long period to allow nuclear interactions to take place, so that there is a net release of energy. Detailed calculations show that the break-even condition can be expressed as the Lawson criterion: ni > 101** cm"3 s , where n is the particle density (cm"3) and T is the confinement time (seconds). The scientific feasibility of nuclear fusion as an energy source thus requires heating a plasma to a temperature of ~20 keV and achieving a particle confinement time greater than that given by the Lawson criterion. 2.2 Confinement and Heatinc Schemes The basic confinement and heating conditions may be met, in principle, In many ways. In some systems, such as the stellarator, these two conditions may be satisfied more or less independantly; ?n the 0-pinch they are inextricably connected. The Lawson criterion allows a wide range of densities to be used in achieving fusion conditions; we may use th.s fact to classify the various confinement schemes into two broad categories: Low density (n~ 101* -1017 cm"3 T Z 1 - 10"3s) High density (n~1020 -1026 cm"3 T Z 10"6 - 10"12 s) . - 3 - 2.2.1 Low Density Systems The low density systems can again be divided according to two criteria: the magnetic field configuration - open or closed - and the ratio, (3, of plasma pressure to magnetic field pressure The major low density confinement systems are classified according to these two criteria in Table i. \« - nkT Low (< 0,1) High (> 0.1) Confining ^v field X. Open Mirror machine Straight 6-pinch Closed Stellarator, Diffuse pinch. torsatron Toroidal 6-pinch, Tokamak high-B stellarator Internal conductor Astron Table I Major types of low density confinement systems Mirror machine: The simple magnetic mirror system is macroscopically unstable against the magnetohydrodynamic (MHD) instability. Stability has been achieved against this instability by creating a "minimum-B1' configuration In which the magnetic field increases in all directions away from the central plasma region. Plasma production and heating have been achieved mainly by energetic particle injection, adiabatic compression, and r.f. heating. The major difficulty in these devices is the loss of particles along the magnetic field lines, through the mirrors. This may have two serious consequences: (a) the loss of energetic particles may represent too high a loss rate in a reactor system; - i» - (b) The non-Maxwellian nature of the resulting velocity distribution excites a variety of microscopic instabilities which may prevent the achievement of the required confinement times. Straight 0-pinch: in the 6-pinch, a rapidly rising axial magnetic field (B ) induces a surface current (L) in a low density pJasma,, The resultant jflB force rapidly compresses and heats the plasma, Confinement is achieved by the high axial magnetic field. The major loss mechanism is axial diffusion. Reactor conditions would require a length of several kilometres and very large electrical power output (1010 - 1011 watts). Stellarator, torsatron: ^A in the stellarator and related toroidal devices, particularly the torsatron, containment of the plasma is achieved by a magnetic field of complex geometry, consisting of closed nested toroidal surfaces, produced entirely by currents flowing in external conductors In the case of the stellarator, toroidal coils producing a large toroidal field, B , and helical windings produce the required field configuration. In the torsatron the required field configuration can be produced In a single set of variable pitch helical windings. A major feature of the stellarator is that heating and confinement are separated functions Thus, stellarator plasmas have been heated in a variety of ways, The most important of these, from the reactor point of view, are: (aj Neutral particle injection — a beam of energetic neutral particles is injected into the plasma (as is done in the mirror machines); (b) Turbulent heating — large currents induced in the plasma create sma'i scale turbulence which aids in the transfer of energy to the plasma; (c) Radio frequency heating — plasmas exhibit a number of natural resonances and energy transfer from waves to particles can be very efficient in the vicinity of some of these resonances. Tokamak: i.»Cf^ • -vrccoJ in the Tokamak the plasma is both heated and confined by currents flowing parallel to the major circumference of the torus. The self magnetic field, BQ, of this current, an externally-generated toroidal field, B , and - 5 - induced wal currents, or a small magnetic field parallel to the major axis of the torus, produce the required field configuration. Supplementary heating will probably be required: this may be achieved by any of the methods used for stellarator heating, in addition, adiabatic compress1on of the plasma, in principle, can be accomplished very simply and economically by moving the plasma (using the plasma current and a small transverse magnetic field) to a position of smaller major diameter; this moves the plasma to a region of higher magnetic field (because of the radial dependence of the large toroidal field) , thus compressing and heating it, interna^ conductor systems: Systems with internal current-carrying conductors are unlikely to be of interest for a reactor. However, they continue to provide valuable Information on plasma confinement and stability in easily variable magnetic field configurations. Astron: in the Astron a relativistic charged particle layer creates a dosed- Hne magnetic field configuration which !s capable, in principle, of heating and confining a plasma at reactor conditions. Currently, research is being conducted with relativistic electrons; favourable results would justify serious consideration being given to this approach. Diffuse pinch: in the Tokamak and stellarator the poloidal field is very much smaller than the toroidal field (B„/B ~ 0.1) and the 3-value is relatively o Z smallo A large expenditure is therefore required to produce the toroidal field. A more economically attractive approach (at least in this regard) is the general class of high-3 devices. The diffuse pinches, screw pinches, and belt pinches, are variants of configurations that are Mhigh-&, current carrying toroidal devices". In these devices Bfl~B -- the magnetic field y z has a very high shear and 3 may be as high as ~0.5. Zeta was the first major device of this type. — ^^u»«^ "r1"? Plasma heating in these devices is achieved by the large axial currents or by fast compression. - 6 - Toroidal 9-pinches, high-8 stellarators: The stellarator configuration is limited to very low (3-values. It may however be possible to achieve a stable configuration, at higher B, using more complex field configurations and, possibly, feedback stabilization. Examples of such devices are ISAR T-1 (Garching) which is a toroidal 6-pirich in which a combination of helical windings U=1 and £=2) may be able to provide an equilibrium configuration, in a similar, and larger device (SCYLLAC), the Los Alamos group is investigating feedback stabilization on a toroidal sector. Other approaches: This rather oversimplified classification scheme omits a number of possible devices.
Details
-
File Typepdf
-
Upload Time-
-
Content LanguagesEnglish
-
Upload UserAnonymous/Not logged-in
-
File Pages26 Page
-
File Size-