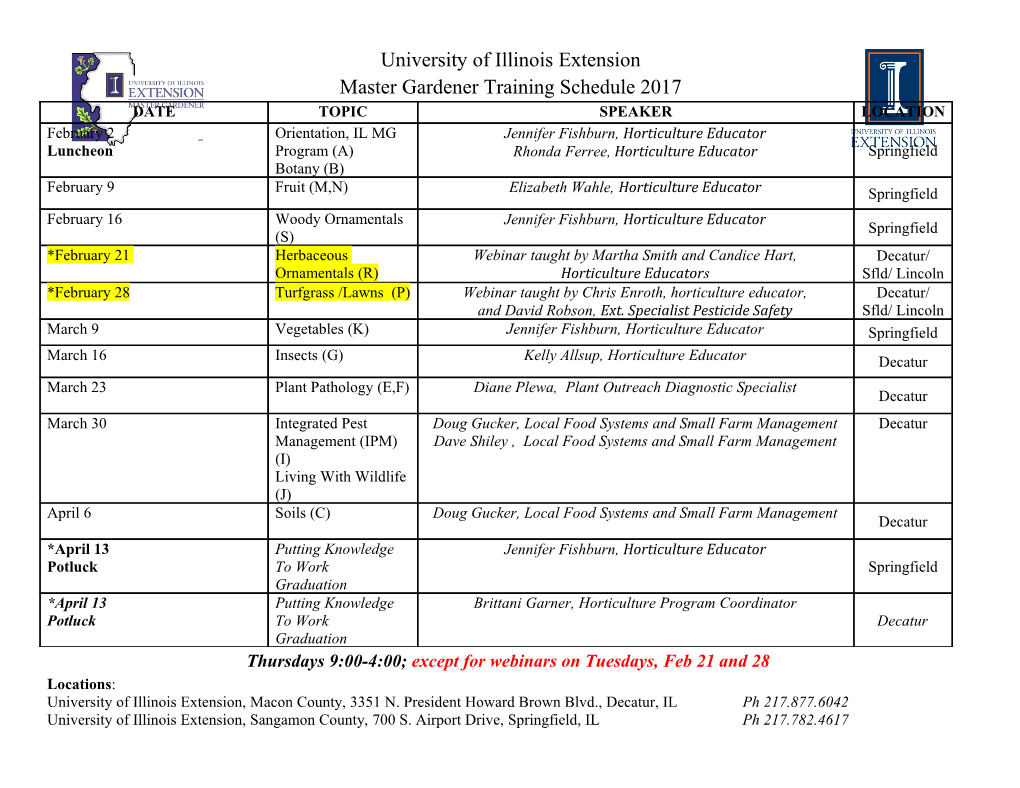
Downloaded from http://cshperspectives.cshlp.org/ on September 29, 2021 - Published by Cold Spring Harbor Laboratory Press Design Principles of Phosphorylation- Dependent Timekeeping in Eukaryotic Circadian Clocks Koji L. Ode1,2 and Hiroki R. Ueda1,2 1Department of Systems Pharmacology, Graduate School of Medicine, The University of Tokyo, Hongo 7-3-1, Bunkyo-ku, Tokyo 113-0033, Japan 2Laboratory for Synthetic Biology, RIKEN Quantitative Biology Center, 1-3 Yamadaoka, Suita, Osaka 565-0871, Japan Correspondence: [email protected] The circadian clock in cyanobacteria employs a posttranslational oscillator composed of a sequential phosphorylation–dephosphorylation cycle of KaiC protein, in which the dynamics of protein structural changes driven by temperature-compensated KaiC’s ATPase activity are critical for determining the period. On the other hand, circadian clocks in eukaryotes employ transcriptional feedback loops as a core mechanism. In this system, the dynamics of protein accumulation and degradation affect the circadian period. However, recent studies of eu- karyotic circadian clocks reveal that the mechanism controlling the circadian period can be independent of the regulation of protein abundance. Instead, the circadian substrate is often phosphorylated at multiple sites at flexible protein regions to induce structural changes. The phosphorylation is catalyzed by kinases that induce sequential multisite phosphorylation such as casein kinase 1 (CK1) with temperature-compensated activity. We propose that the design principles of phosphorylation-dependent circadian-period determination in eukary- otes may share characteristics with the posttranslational oscillator in cyanobacteria. he circadian clock produces near-24-h which strains with a circadian period matching Trhythms in physiological activities. From the light–dark cycle of their environment be- multicellular animals to plants, circadian clocks came dominant in the population (Woelfle regulate cellular metabolic pathways and other et al. 2004). This advantage should apply to physiological responses of the organism, such as many species; circadian rhythmicity of physio- sleep–wake cycles and immune responses (Levi logical activities is observed in evolutionarily and Schibler 2007; Bass and Takahashi 2010; distant species. Conserved features of the mo- Mohawk et al. 2012; Albrecht 2013; Greenham lecular mechanisms that drive circadian clocks and McClung 2015; Lu et al. 2017; Sanchez and in different phyla have been revealed through Kay 2017). The evolutionary advantage of a cir- studies in a variety of organisms, including con- cadian clock was demonstrated by coculture ex- ventional models such as rodents in mammals, periments with prokaryotic cyanobacteria, in Drosophila melanogaster in insects, Arabidopsis Editors: Paolo Sassone-Corsi, Michael W. Young, and Akhilesh B. Reddy Additional Perspectives on Circadian Rhythms available at www.cshperspectives.org Copyright © 2018 Cold Spring Harbor Laboratory Press; all rights reserved; doi: 10.1101/cshperspect.a028357 Cite this article as Cold Spring Harb Perspect Biol 2018;10:a028357 1 Downloaded from http://cshperspectives.cshlp.org/ on September 29, 2021 - Published by Cold Spring Harbor Laboratory Press K.L. Ode and H.R. Ueda thaliana in higher plants, Neurospora crassa in TRANSCRIPTION NEGATIVE FEEDBACK IN fungi, and Synechococcus elongatus in cyano- EUKARYOTIC CIRCADIAN OSCILLATORS bacteria. In all organisms, circadian rhyth- micity is cell-autonomous. Even in mammals, Figure 1 summarizes the major molecular com- various cell types show robust circadian rhyth- ponents of circadian oscillators in different phy- micity in gene expression and physiological ac- la. Eukaryote oscillators have conserved negative tivity, even when cultured in vitro (Welsh et al. feedback loops in the transcription–translation 1995; Yagita et al. 2001; Nagoshi et al. 2004; network (Fig. 1A). The PERIOD protein (PER) Yoo et al. 2004). Cell-autonomous circadian is a key transcriptional repressor in mammals oscillation is produced by the system-level or- and Drosophila. PER binds and inhibits a basic chestration of transcription–translation feed- helix–loop–helix transcription-activator com- back and/or a posttranslational interaction net- plex consisting of CLOCK and BMAL1 in mam- work (Gallego and Virshup 2007; Ukai and mals (Fig. 1B). In Drosophila, PER inhibits a Ueda 2010; Millius and Ueda 2017), but the complex of CLOCK (CLK) and CYCLE (CYC), molecular components of the oscillators espe- a BMAL1 ortholog (Fig. 1C). The CLOCK– cially for transcriptional or translational regu- BMAL1 (CLK-CYC in Drosophila) complex ac- lators differ between species (Rosbash 2009; tivates Per transcription; thus, the overall tran- Brown et al. 2012; van Ooijen and Millar scription network creates a negative feedback 2012; Takahashi 2017). However, the question loop. PER binds other partners in various organ- arises whether a common design principle un- isms to act on transcription activators. In Dro- derlies the molecular components of circadian sophila, PER represses its own transcription by clocks in different organisms. binding TIMELESS (TIM), the vertebrate ho- In most organisms, circadian molecular os- molog of which maintains chromosomal integ- cillators seem to be based on transcription– rity (Chou and Elledge 2006; Tanaka et al. 2009). translation feedback loops (TTFLs). However, In contrast, mammalian PER forms a complex how circadian clocks maintain a robust 24-h with CRYPTOCHROME (CRY). The crypto- oscillation is a mystery. The suprachiasmatic chrome superfamily is widely conserved from nucleus, a region of the brain central to circadi- bacteria to plants, but nonmammalian crypto- an rhythm in mammals, can sustain oscillating chrome functions as a blue-light receptor rather gene expression for years when cultured in vitro than as a circadian transcription repressor (Lin (Yamazaki and Takahashi 2005). However, the and Todo 2005; Ozturk et al. 2007). Phylogenet- periodic gene expression in artificial biological ically, the closest homolog of mammalian cryp- oscillators encoded as TTFLs is less stable when tochrome is 6–4 photolyase, which catalyzes the subjected to cell-intrinsic or cell-extrinsic repair of damaged DNA residues using energy changes (Elowitz and Leibler 2000; Danino from blue light. These functional divergence of et al. 2010). This observation suggests that an TIM and CRY in maintaining DNA integrity additional layer exists in the period-keeping and circadian rhythmicity may be related to mechanism of circadian cellular oscillators. the evolutionarily close relationship of DNA- Here, we review the circadian-clock features in damage responses and circadian clocks in an various organisms from the perspective of environment with daily oscillations in sunlight. TTFL-based design principles and posttransla- The molecular components of circadian tional modifications of oscillator components. negative feedback loops differ between fungi Through the comparison between a phosphor- and plants. White Collar complex (WCC) and ylation-based oscillator in cyanobacteria and FREQUENCY (FRQ) are the primary drivers of multisite phosphorylation of clock components Neurospora circadian clocks (Fig. 1D). WCC ac- that can regulate the circadian period in eukary- tivates FRQ transcription, which in turn inhibits otes, we propose that shared design principles WCC and creates the negative feedback loop. can be found in the circadian period-determi- The canonical core feedback architecture of nation mechanisms. the circadian clock in plants is more complex 2 Cite this article as Cold Spring Harb Perspect Biol 2018;10:a028357 Downloaded from http://cshperspectives.cshlp.org/ on September 29, 2021 - Published by Cold Spring Harbor Laboratory Press Phosphorylation-Dependent Timekeeping in Eukaryotes ABCCanonical design principle Mammals Fruit fly P P P CRY P TIM P X P PER P PER P X gene BMAL1 Per gene CYC per gene CLOCK Cry gene CLK tim gene DEFungi Higher plants P LHY P FRQ P CCA1 P EC PRRs TOC1 WC1 frq gene CCA1 gene WC2 LHY gene Figure 1. Shared structure of transcription–translation feedback loop (TTFL)-based eukaryotic circadian clocks. (A) Diagram showing the basic structure of a circadian negative-feedback transcription oscillator. The transcrip- tion repressor is regulated by multisite phosphorylation and proteasome-mediated proteolysis. (B–E) Major components of the circadian TTFL-based oscillator in each species. Note that the indicated components and pathways were selected based on the context of this review; the actual circadian systems are far more complex reaction networks involving numerous gene products. EC (evening complex) composed of ELF4, LUX, and ELF3. (Fig. 1E). In Arabidopsis, the canonical model period of TTFL-based oscillators is determined involves transcription feedback loops around through the dynamics of protein quantity: the two transcription repressors, CCA1 and LHY, repression phase is initiated by the accumula- which suppress the transcription of both activa- tion of transcription repressor(s) and ceases tors and inhibitors of CCA1/LHY expression, when the repressors have disappeared. Indeed, creating an architecture combining positive the circadian period is reported to be regulated and negative feedback. through the delayed timing (phase) of the accu- Theoretical studies emphasize the impor- mulation of mammalian transcriptional repres- tance of a negative feedback loop encoded as a sor Cry messenger RNA (mRNA) (Ukai-Tade-
Details
-
File Typepdf
-
Upload Time-
-
Content LanguagesEnglish
-
Upload UserAnonymous/Not logged-in
-
File Pages19 Page
-
File Size-