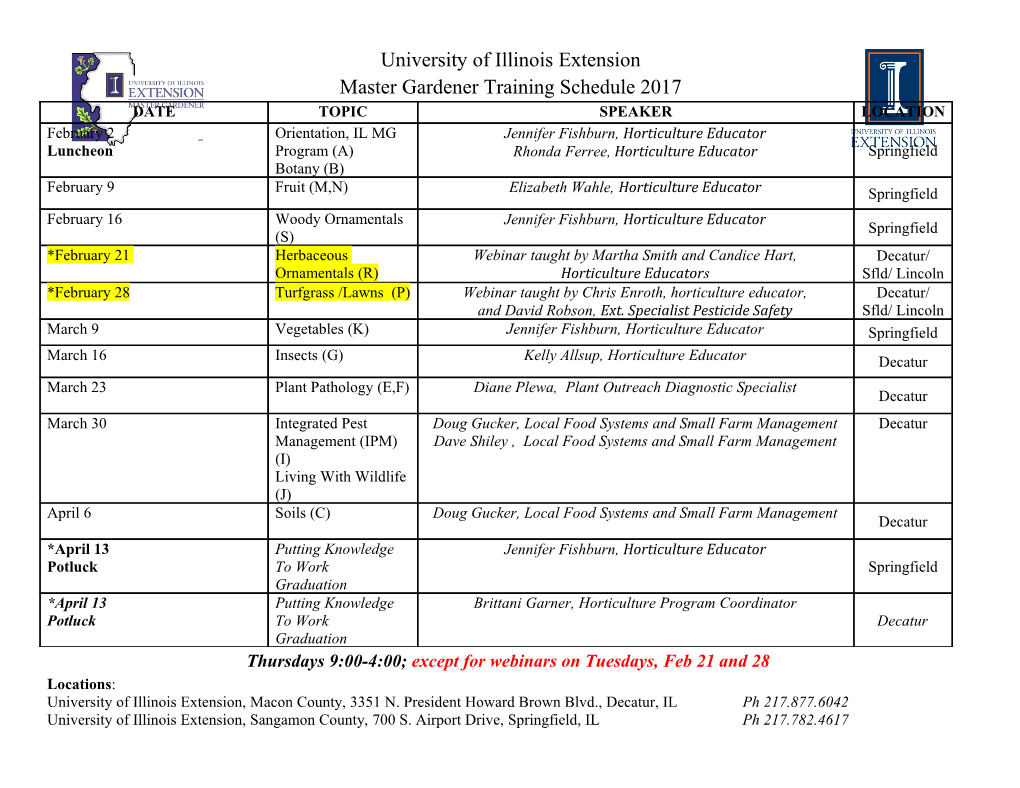
Effect of Caffeine Exposure on Gene Expression in Human Mesenchymal Stem Cells Madison Pedreira 1 Abstract Millions of pregnant women consume caffeine during their pregnancy, which may end up affecting their child’s development. In animal models, fetal caffeine exposure has shown to alter gene expression and embryonic cardiac tissue while also producing long-term effects. Some human studies have shown miscarriage and reduced birth weight to be an effect of fetal caffeine exposure, but there are differing opinions. To better understand the influence of caffeine on stem cell gene expression, we cultured mesenchymal stem cells (MSCs) from human umbilical cords. One group of samples were obtained from mothers that consumed high levels of caffeine during their pregnancy and the other group consumed lower caffeine levels. RNA was isolated from 3 cell lines for both levels of caffeine exposure and analyzed by RNAseq. Analysis of RNAseq data revealed differences in gene expression between the two groups. In this report, we demonstrate that genes involved in apoptosis, gliomas, and transcriptional activation are upregulated in the high caffeine samples, while genes involving apoptosis inhibition, embryonic and neural development, and transcriptional repression are downregulated. 2 Introduction Environmental factors can have a major effect on the development of the fetus in the womb. Anything consumed by the pregnant mother has the potential to affect the fetus’s gene expression directly or through epigenetic pathways[1]. It has been demonstrated that smoking or drinking during pregnancy can have serious consequences on the fetus, however, caffeine has not been considered as dangerous. Understanding the consequence of caffeine consumption during pregnancy is a public health concern that directly affects our children and their development. Animal studies have shown ventricular septal defects[2] and decreased embryonic cardiac tissue[3] to be heart-related abnormalities during embryonic development when exposed to caffeine in utero. Caffeine exposure has been correlated with lower birth weight[4] and miscarriage in human babies[5], but these concerns are not shown by all researchers. However, data from our previous experiments in animals showed that embryonic caffeine exposure leads to altered cardiac function in mice[3, 6, 7], as described in the following paragraphs. The mouse data we have collected already is important for us to consider when comparing our human stem cell analysis in order to better understand the effect of fetal caffeine exposure. One of our previous animal studies showed that in utero exposure to caffeine affects embryonic development and cardiac function after birth[3]. After pregnant mice were treated with caffeine, either the embryos were collected, or the pregnant mothers gave birth. This allowed for both the analysis of prenatal fetus and the postnatal offspring to understand the extent to which caffeine affects post-fertilization development. In the embryos, cardiac histology was examined and showed that the caffeine-treated group had a smaller cross-sectional area of the ventricular myocardium, indicating that the embryonic development of the heart was negatively affected by caffeine exposure. In the pups, echocardiography was used to determine cardiac function, showing a decrease in overall cardiac function and in systolic function. This information indicates that in utero caffeine exposure in mice affects both the fetal development before birth and the offspring development after birth in the heart, which is a concern that must be studied in human samples to determine if caffeine consumption during pregnancy is dangerous. A relevant concern is that fetal caffeine exposure may affect the offspring and generations after that. The consequence of consuming caffeine becomes much greater if this is true, and another one of our previous animal studies indicates that this may be the case[7]. Pregnant mice were treated with caffeine, and their offspring and the next two generations displayed altered cardiac morphology and function. Generation 1 was the only generation exposed to caffeine in utero, yet the next two generations displayed adverse effects into adulthood. Symptoms of altered cardiac function often did not show until adulthood of the 2nd and 3rd generations, indicating that transgenerational fetal caffeine exposure may have latent effects. This raises questions about how representative this study is to humans. In order to understand the extent of the danger of caffeine consumption during pregnancy, these findings must be confirmed in human models. These findings are also supported in animal cell studies. Another previous study demonstrates that gene expression and cell morphology are altered when mouse cardiomyocyte cells are treated with caffeine[6]. These results showed that the cells treated with caffeine had higher mRNA expression in several cardiac structural genes, cardiac transcription factors, and cardiac 3 miRNAs. The cells also displayed morphological changes and reduced in numbers after a certain concentration of caffeine was administered. This study supports the results from the last two studies discussed here, but with cellular data. Using cells to study the effect of caffeine treatment is helpful because there is less genetic variability that may affect the results, as cells grow in a more controlled environment than breeding mice. As described, there is existing evidence in mouse studies that indicate that in utero caffeine exposure has effects on embryonic development that may lead to altered phenotypes in adulthood. The next step in this sequence of studies is to use a human model to verify the data we have from animal studies. The model for fetal development in this study is human umbilical cord MSCs taken from subjects, and the samples were separated into two groups, high vs. low in utero caffeine exposure. High caffeine exposure is defined as greater than 71.3 mg/day (about 6 oz. coffee) and low caffeine exposure is defined as less than 24.9 mg/day. This is not an ideal model, but it is a good first step because it allows us to examine human fetal cells in response to in utero caffeine exposure. Because these cells are mesenchymal stem cells from fetal tissue, we can compare them to our mouse data. MSCs are undifferentiated stem cells and will allow us to identify genes that are differentially expressed in response to caffeine exposure. This will give us a basis to understand these cells so that future studies can be done on these cells after differentiation into cardiomyocytes. RNAseq data from each human sample group (high vs. low caffeine exposure) will be compared on the basis of gene expression to determine any differences between the two groups. This also allows us to determine if human MSCs respond to caffeine similarly as we have observed in animal models. Here, we use an RNAseq analysis pipeline to understand the differentially expressed genes between high caffeine and low caffeine groups. This will help us understand if caffeine consumption during pregnancy has an effect on gene expression in the embryo, and whether this has long-term effects on the health of the fetus. 4 Methods Quality control and alignment to transcriptome The 6 RNAseq samples (3 high caffeine, 3 low caffeine) were analyzed for quality control using FastQC (version 0.11.4)[8] and MultiQC (version 1.1)[9], which was done before and after trimming. This provides quality scores, per base sequence content, and GC content. The sequences were trimmed using Trimmomatic (version 0.36)[10] based on the quality control report to remove low quality bases. The samples were aligned to the human transcriptome using STAR (version 2.7.3a)[11]. STAR is an efficient alignment tool for RNAseq because it aligns transcripts and takes splicing into account instead of mapping to the genomic sequence. Quantification and Differential Expression analysis Gene and transcript abundance and expression values were quantified with RSEM (version 1.2.31)[12]. RSEM also produces a correlation scatterplot with correlation scores between each replicate, along with a Multi-Dimensional Scaling (MDS) plot for the raw expression data. DESeq2[13] was used for the differential expression analysis to determine which of the genes identified by RSEM were differentially expressed between the two study conditions. The significant genes were filtered to only include log2(FC) ≥ |1.0| and False Discovery Rate corrected p-value ≤ 0.05. Another MDS plot is generated in DESeq2 once the expression data is normalized. DESeq2 filtered protein-coding genes specifically so that the genes of interest may be identified in developmental pathways to understand the effect that caffeine has on embryonic development. Pathway identification GeneCards is a searchable human gene database available on the internet and was used to identify potential pathways in the differentially expressed genes found in the RNAseq pipeline. A more accurate and robust pathway analysis would be done in the continuation of this study, but GeneCards was used here as a preliminary pathway analysis for the genes found. GeneCards pulls information from Entrez and UniProt to include in their summary for each gene. 5 Results and Discussion Quality control analysis shows high quality scores and transcriptome alignment rate In order to better understand the quality of the sequencing of our isolated RNA samples, we must first run quality control before any further analysis. Quality control provides the information that dictates trimming, which will lead to a better alignment to the transcriptome. The quality of the samples was analyzed using FastQC[8], and the quality report determined how much needed to be trimmed off the ends of our sample to ensure that only high quality bases are retained. This will allow for the closest possible mapping to the transcriptome, in which we used STAR[11]. Here, the percentage of reads retained after trimming (Table 1) is high due to the high quality scores of the samples. The quality control used here assigns only 4 quality score values as compared to the previous range of 40, resulting in the highest possible scores for these samples.
Details
-
File Typepdf
-
Upload Time-
-
Content LanguagesEnglish
-
Upload UserAnonymous/Not logged-in
-
File Pages16 Page
-
File Size-