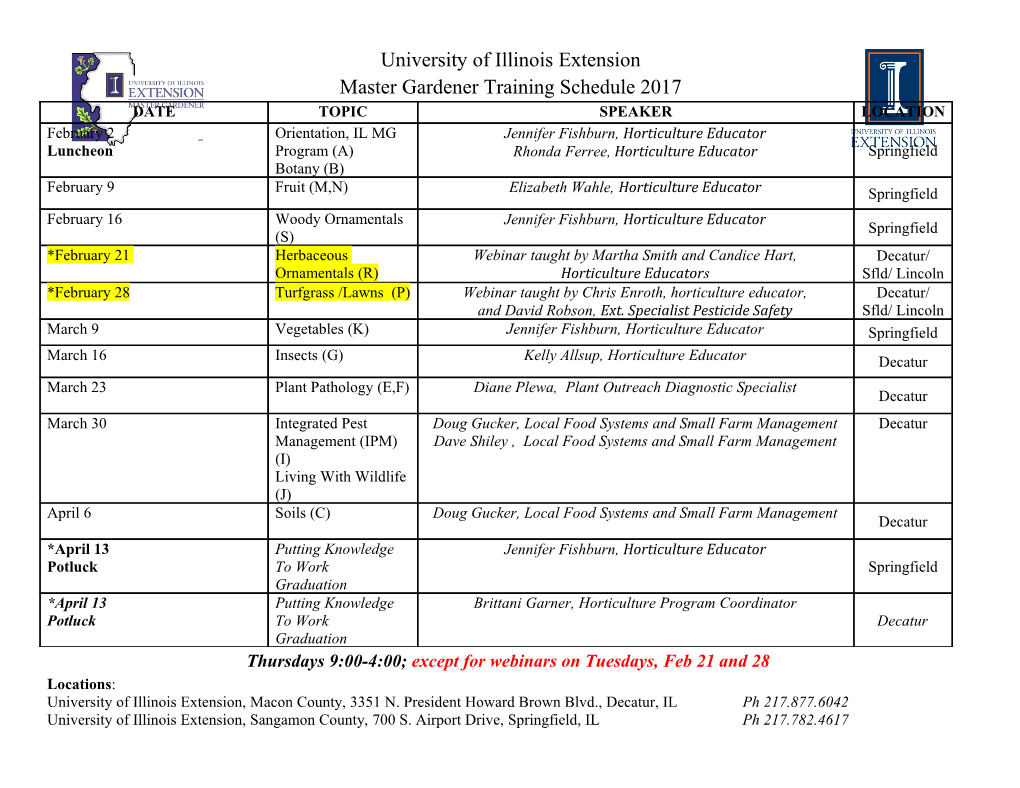
Groundwater Management: Quantity and Quality (Proceedings of the Benidorm Symposium, October 1989). IAHS Publ. no. 188,1989. A groundwater management strategy for salinity mitigation in the Victorian riverine plain, Australia R.S. EVANS & J. NOLAN Rural Water Commission, 590 OrrongRoad, Armadale, Victoria, 3143, Australia Abstract The major salinity problems emerging in the Riverine Plain of Northern Victoria are described. The long term viability of agriculture in this region is now threatened by land salinization and waterlogging; a direct result of increased rates of recharge to groundwater in response to clearing of natural vegetation and intensive irrigation. Without large scale dewatering, the groundwater system is unable to dissipate the increased recharge, hence water tables will continue to rise and exacerbate the existing problem. A groundwater management strategy, based on encouraging groundwater development is described. The principle constraints on such development, the need for control of aquifer salinity and salt export, are presented in the context of an overall conjunctive use policy. Specific strategy initiatives to encourage an appropriate and sustainable level of groundwater use are presented. Une stratégie d'exploitation des nappes aquifères pour la réduction de la salinité dans la plaine riveraine de Victoria, en Australie Résumé Cet article présente les principaux problèmes de salinité de la plaine riveraine, au nord de Victoria, en Australie. Aujourd'hui, dans cette région, la viabilité à long terme de l'agriculture est menacée par la salinisation et la saturation des terres et des eaux. Ce fait est l'une des conséquences de l'augmentation des recharges de nappes aquifères causée par l'élimination de la végétation naturelle et par des irrigations intensives. Si cette eau en excédent n'est pas évacuée à grande échelle, le système aquifère ne peut pas dissiper les eaux excédentaires ; les nappes d'eaux souterraines continueront donc à monter et aggraveront le problème actuel. Une stratégie d'exploitation, basée sur l'incitation au développement des nappes d'eaux souterraines, est décrite dans cette étude. Les principales restrictions de tels développements, le besoin de contrôle de salinité aquifère et d'exportation de sel sont compris dans un plan d'action d'usage général et conjoint. Des initiatives de stratégies spécifiques, dont le but est d'encourager un niveau 487 R.S. Evans & J. Nolan 488 approprié et pouvant être maintenu longtemps de l'emploi des nappes d'eau, sont aussi présentées, INTRODUCTION A groundwater management strategy for the Victorian Riverine Plain (Fig. 1) which aims at addressing the serious and rapidly expanding salinity problems of the region is presented. This strategy is only one component of the regional land and water salinity management strategy which is currently in preparation. The groundwater management component primarily applies to private groundwater development for on-farm use. The groundwater strategy centres on the development and careful management of the better quality groundwater resources in Northern Victoria. Recharge reduction, a major component of the salinity strategy, is not covered in this paper. Fig. 1 Riverine Plain of northern Victoria. EXISTING SITUATION Regional perspective The Murray Basin in southeastern Australia represents a large predominantly closed groundwater basin of approximately 320 000 km2. Tertiary and Quaternary sediments are typically 300 m thick and form an extensive sequence of alternating aquifers and aquitards. Recharge occurs 489 Groundwater management for salinity mitigation from both point sources around the basin rim (predominantly by river recharge) and by diffuse recharge through vertical infiltration over much of the region. Discharge occurs over large areas of the basin through évapotranspiration from shallow watertables, by discharge to the River Murray and at concentrated discharge regions where groundwater outcrops to form extensive saline lake systems. The Murray Basin is divided on geomorphic and hydrogeological grounds into the riverine plain to the east, comprising predominantly good to moderate quality groundwater (500 to 3000 mg/1) and non marine sediments, and the Mallee in the west, comprising predominantly marine sediments with moderate to poor quality groundwater (10 000 to 60 000 mg/1). Increasing land and stream salinization within the riverine plain is largely attributed to land and water management changes since European settlement, some 150 years ago. The two primary changes, the widespread clearing of native vegetation, and replacement with shallow rooted pastures, and the introduction of predominantly surface water based irrigation, have led to a dramatic increase in recharge and subsequent rise in shallow watertables and deep aquifer pressures. The rising water level has mobilised salts (predominantly sodium chloride) stored in the previously unsaturated profile, and transported them towards the surface where evaporative concentration occurs. Stream salinities have increased through surface run-off of the concentrated salts and increased base flow. In general, the rising groundwater levels are continuing at the rate of 0.05 to 0.1 m/year. Large tracts of both dryland (non irrigated) and irrigated pasture are threatened or already severely affected by saline groundwater and/or waterlogging. Hydrogeological framework Three major stratigraphie units comprise the riverine plain. The uppermost unit, the Shepparton Formation, can be idealized as an extensive silt and clay sheet intersected by randomly distributed interconnected sand beds (prior streams) of variable salinity. The watertable level is influenced by leakage to or from the underlying Calivil Formation and Renmark Group, the "Deep Lead", which from the regional perspective may be considered to be a single highly transmissive sand and gravel aquifer of good quality water (Fig. 2). The estimated recharge over the region varies from around 10 mm/year in the highlands up to 170 mm/year beneath heavily irrigated sandy soils. On average, in the irrigated areas it is around 30 mm/year. The shallow watertable in the Shepparton Formation tends to be between 10m and 1 m below the surface. Where the watertable is below the zone of évapotranspiration (i.e. deeper than about 2 m) it is generally exhibiting a steady rise, but significantly influenced by local features. In the eastern riverine plain, the "Deep Lead" generally has a potentiometric RS. Evans & J. Nolan |H! 200 -2000 mg/l | ] 2000 - 6000 mg/l f///| 6000 - 30000mg/t Fig. 2 Groundwater quality (a) Shepparton Formation, (b) Deep Lead. surface below that of the Shepparton Formation, hence leakage is downwards. However, the "Deep Lead" potentiometric surface is rising at a rate of up to 0.2 m/year (Macumber, 1978) and thus commonly will equal or exceed the shallow watertable within a few decades if it is not managed through controlled pumping to maintain vertical drainage of the overlying Shepparton Formation. In the western part of the riverine plain the deeper aquifer is generally artesian. Current water allocation and usage practices Surface water Extensive predominantly surface water based irrigation exists over much of the riverine plain. A complex channel system exists to distribute the water (typically 1.6 x 10 Ml/year) from dams located in the highlands. The irrigation system commenced in the 1880s and has undergone significant expansion up to the 1960s. Surface water entitlements to individual farms (with an average size of 70 ha) have been based on 6 Ml/ha Groundwater All bores, other than those for domestic and stock purposes, in Victoria require a licence to extract groundwater. A maximum volume of water able to be extracted is a condition of the licence. Within the Victorian riverine plain there are approximately 950 groundwater bores licenced to extract 255 000 Ml/year for irrigation purposes (and many thousands of domestic and stock bores). The irrigation licences consist of about 750 shallow systems (less than 30 m deep multiple spear points) and 200 deep bores (typically 60 to 100 m deep). Over 70% of groundwater extraction occurs from bores installed at depths of less than 20 m. The actual irrigation groundwater usage and the authorised usage over time is shown in Fig. 3. 491 Groundwater management for salinity mitigation The usage has increased from an estimated 4500 Ml in 1974 to 108 000 Ml in 1986. The actual groundwater usage is about 40% of the authorised volume in a normal year rising to about 80% in a drought year, as observed during 1982 and 1983. Currently groundwater usage is approximately 8% of the applied irrigation water across the region. 250- £200 AUTHORIZED LICENCED 150- ALLOCATION UJ 100- < ESTIMATED ACTUAL USAGE —i 1 1 1 82 83 84 85 1986 Fig. 3 Authorised and historical groundwater use. Irrigation bores are primarily installed to provide additional water for irrigation, either as insurance against drought or to provide a long term supplementary source of water to the channel-supplied surface water. Groundwater bores are rarely, if ever, installed by the public primarily for salinity or water logging control. In addition to private groundwater usage, there is substantial public-scale groundwater pumping for watertable and salinity control purposes. There are 79 pumping installations in the eastern region protecting high value horticultural and pasture (18 000 ha) areas and another six pumps directed at pasture protection. Almost all the groundwater pumped is discharged to surface water drains and channels where approximately 60% is used for irrigation and 40% is discharged to the River Murray- Groundwater salinity is generally about 2600 mg/1. In the order of 10 900 Ml/year are pumped and hence 11 300 tonnes of salt enter the River Murray per year. Land and stream salinisation Rising groundwater levels across the Victorian riverine plains are evident beneath the irrigation regions and the adjacent dryland areas. It is estimated KS. Evans & J. Nolan 492 that salinity is costing approximately $50 million per year as lost agricultural production within the irrigated areas; about 140 000 ha have suffered from losses in agricultural productivity as a result of salinity.
Details
-
File Typepdf
-
Upload Time-
-
Content LanguagesEnglish
-
Upload UserAnonymous/Not logged-in
-
File Pages14 Page
-
File Size-