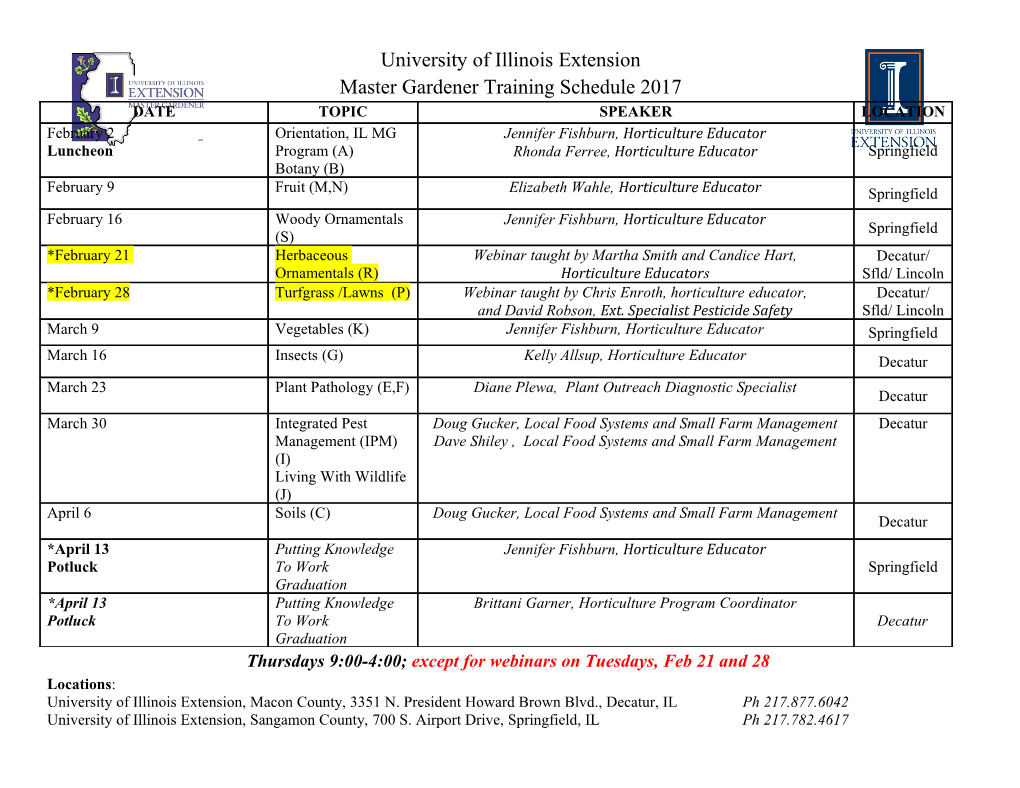
Structure of exoplanets David S. Spiegela,1, Jonathan J. Fortneyb, and Christophe Sotinc aSchool of Natural Sciences, Astrophysics Department, Institute for Advanced Study, Princeton, NJ 08540; bDepartment of Astronomy and Astrophysics, University of California, Santa Cruz, CA 95064; and cJet Propulsion Laboratory, California Institute of Technology, Pasadena, CA 91109 Edited by Adam S. Burrows, Princeton University, Princeton, NJ, and accepted by the Editorial Board December 4, 2013 (received for review July 24, 2013) The hundreds of exoplanets that have been discovered in the entire radius of the planet in which opacities are high enough past two decades offer a new perspective on planetary structure. that heat must be transported via convection; this region is Instead of being the archetypal examples of planets, those of our presumably well-mixed in chemical composition and specific solar system are merely possible outcomes of planetary system entropy. Some gas giants have heavy-element cores at their centers, formation and evolution, and conceivably not even especially although it is not known whether all such planets have cores. common outcomes (although this remains an open question). Gas-giant planets of roughly Jupiter’s mass occupy a special Here, we review the diverse range of interior structures that are region of the mass/radius plane. At low masses, liquid or rocky both known and speculated to exist in exoplanetary systems—from planetary objects have roughly constant density and suffer mostly degenerate objects that are more than 10× as massive as little compression from the overlying material. In such cases, 1=3 Jupiter, to intermediate-mass Neptune-like objects with large cores Rp ∝ Mp , where Rp and Mp are the planet’s radius mass. At high and moderate hydrogen/helium envelopes, to rocky objects with masses, for objects that have had time to cool, electron de- roughly the mass of Earth. generacy pressure becomes significant, and the mass/radius re- −1=3 lation changes such that the radius scales as Rp ∝ MP . Note gas giants | hot Jupiters | super-Earths that the H/He comp. curve in Fig. 1 does not display this be- havior at low masses, because this curve is calculated for highly ow can we, from many light years away, learn about the irradiated objects that are a mere 0.045 a.u. from their Sun-like Hinterior structure of exoplanets? Radial velocity observa- star, which prevents them from reaching their zero-temperature tions provide minimum masses of exoplanets; transit observa- radius in 3 billion years (3 Gyr). For cold spheres of H/He, it has tions provide planet radii, and taken singly, neither is especially long been appreciated that the maximum in the mass/radius re- informative about planet structure. However, when we know lation occurs near 4× Jupiter’s mass (9, 10), with a broad peak at both the mass and the radius of a planet we may learn much just over 1 RJ (where RJ is Jupiter’s radius), extending from ∼1/ more about the interior structure. The Kepler satellite (1, 2) is 10th of Jupiter’s mass to ∼100× Jupiter’s mass. For a planet to be a space-based, transit-detecting mission that, as of early 2013, smaller than the H/He minimum radius requires the presence of has identified ∼100 planets and ∼3,000 planet candidates, of a significant heavy-element component, in the form of a core in which the vast majority are almost certainly real (3, 4). Thanks to the object’s center or high-metallicity material well mixed Kepler and ground-based efforts such as the Hungarian Auto- throughout the envelope (where “metals” is taken to mean ele- mated Telescope (HAT) and Super Wide Angle Search for ments heavier than helium). For a planet to be larger than the Planets (SuperWASP) transit surveys (5, 6), there are now more zero-temperature radius (∼1 RJ) requires it to have a high enough than 200 known planets with measured masses and radii, span- temperature that pressure from ions becomes a significant frac- ning a range of irradiation conditions. tion of that due to electrons. Fig. 1 portrays how planet radii relate to masses and incident Before the discovery of transiting planets, it was assumed that fluxes, among the known planets (including the solar system approximately billion-year-old planets—even ones on close-in Kepler Kepler planets) and the candidates [ objects of interest orbits around their stars (so-called “hot Jupiters,” with orbits (KOI)] (7, 8). Several trends are apparent in the data: planets with the largest radii tend to be near Jupiter’s mass and highly Significance irradiated, and Kepler seems to find low-radius planets at a wide range of orbital separations, including some small planets that are extremely highly irradiated. Planets around other stars, or exoplanets, are now known to In the remainder of this paper, we discuss what is known of the be common in our galaxy. Exoplanets span a much wider range structure of the most massive planets (gas giants), of intermediate- of physical conditions than the planets in our solar system, and mass planets (Neptunes), and of low-mass planets (terrestrial include extremely puffy gas giants to compact rocky planets and ocean planets). We conclude by considering how our that can have densities as high as that of iron. The diversity of knowledge of exoplanet structure might improve over course exoplanets allows us to place the planets of our solar system in of the next decade. a broader perspective, and invites further research in the na- scent field of comparative exoplanetology. Here we review the Gas Giants continuum of detected planets, from the gas giants, which are Jupiter and Saturn are essentially giant spheres of hydrogen and composed mostly of hydrogen, to smaller worlds where water helium (H/He) with smaller contributions from heavier elements may provide more of their mass, to rock-iron worlds in some and complex molecules. Many of the known exoplanets have ways similar to Earth. similar mass and similar radius to our local gas giants, and Author contributions: D.S.S., J.J.F., and C.S. designed research, performed research, con- probably have roughly similar bulk structure. The atmosphere, or tributed new reagents/analytic tools, analyzed data, and wrote the paper. weather layer, of such an object is a thin outer region that is of The authors declare no conflict of interest. roughly the same relative depth as the skin of a grapefruit, and is – This article is a PNAS Direct Submission. A.S.B. is a guest editor invited by the Editorial typically defined as the region above the radiative convective Board. boundary,* which can be at pressures on the order of kilobars 1To whom correspondence should be addressed. E-mail: [email protected]. (kbar) for the most strongly irradiated planets and which occurs – in the vicinity of ∼1 kbar in gas giants subjected to lower irra- *The radiative convective boundary is the region bounding the convective interior of – a planet in which heat transport is dominated by convective eddies. This boundary diation, such as Jupiter and Saturn. Below the radiative con- occurs essentially where the vertical temperature gradient becomes subadiabatic and vective boundary, there is a deep envelope extending almost the therefore stable against convection. 12622–12627 | PNAS | September 2, 2014 | vol. 111 | no. 35 www.pnas.org/cgi/doi/10.1073/pnas.1304206111 Downloaded by guest on September 29, 2021 A 2.5 B 2.5 SPECIAL FEATURE 3e+10 25.00 1e+10 Flux (erg Radius vs. Mass 4.600 Mass (M Radius vs. Flux 3e+09 2 1e+09 2 0.855 KOI 3e+08 Solar System 0.157 Solar System / 1e+08 Jupiter cm Planet Planet 3e+07 0.029 ) ) 1.5 2 1.5 1e+07 / s) 0.005 ) 3e+06 Jupiter Jupiter 1e+06 0.001 R[M] for H/He comp. J 1 J 1 R (R R (R 3Gyr old, 0.045AU from Sun S S 0.5 0.5 R[M] for 100% water comp. UN R[M] for Earth−like comp. N U R[M] for 100% iron comp. V E M E V 0 0 M 3 4 5 6 7 8 9 10 11 12 0.001 0.01 0.1 1 10 10 10 10 10 10 10 10 10 10 10 M (M ) Jupiter F (erg/cm2/s) Fig. 1. Radius vs. mass (A) and radius vs. incident-irradiating flux (B) for the confirmed exoplanets, the Kepler candidate planets (KOI), and the planets of our solar system. The ∼200 confirmed planets in this figure are represented with filled large circles; KOI (B) are represented with small white circles; solar system planets are represented with large pentagrams. For comparison, A shows the radius vs. mass relationship for Earth-like composition, which precisely matches the (mass, radius) values for Venus and Earth (26). Planets span masses from less than Earth’stotensoftimesJupiter’s, radii from less than Earth’stomorethandouble − − Jupiter’s, and incident fluxes from nearly zero—in the case HR 8799b, at ∼70 a.u. (27)—to nearly 1012 erg·cm 2·s 1. The planets with the largest radii tend to be close to Jupiter’s mass and highly irradiated. lasting ∼1 week or less)—would have cooled and shrunk to near essentially impossible to learn whether the extremely inflated their asymptotic radius (11, 12). However, the discovery of transiting planets have cores (although, if they do, the larger the core mass, ASTRONOMY hot Jupiters such as HD 209458b (13), with its &1.3 RJ radius, the greater the additional power that is required to explain their suggested that some planets are a good deal puffier than expected radii).
Details
-
File Typepdf
-
Upload Time-
-
Content LanguagesEnglish
-
Upload UserAnonymous/Not logged-in
-
File Pages6 Page
-
File Size-