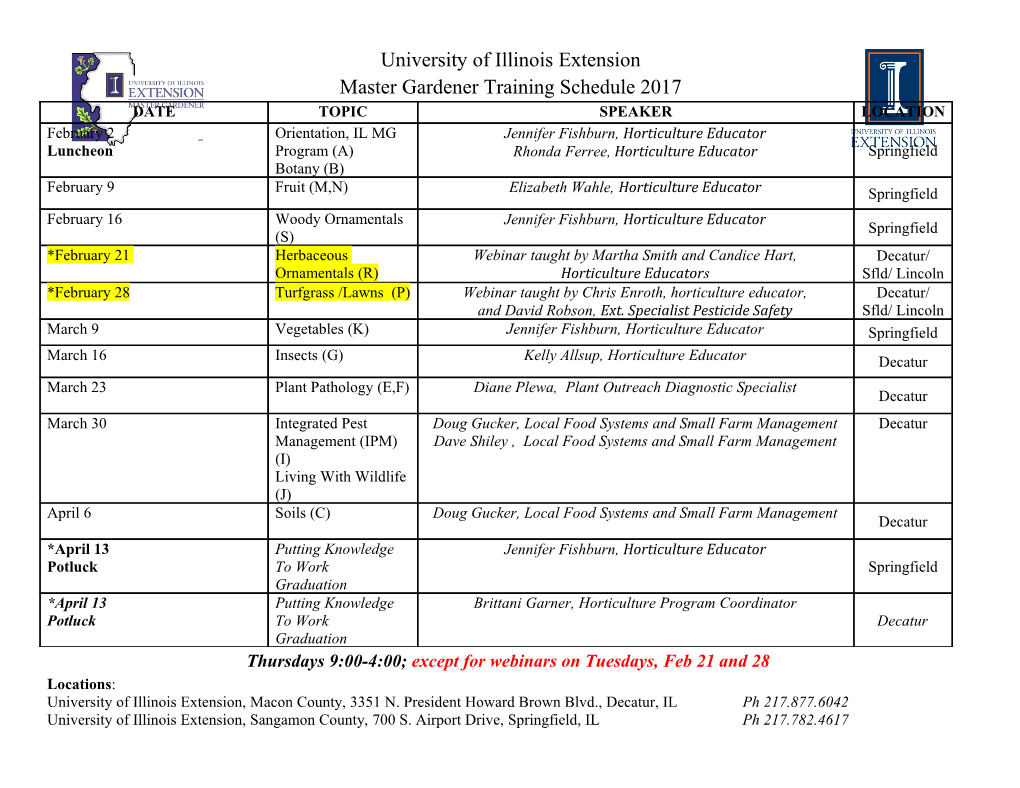
CHAPTER 1 Multiple transport processes in solid oxide fuel cells P.-W. Li, L. Schaefer & M.K. C hyu Department of Mechanical Engineering, University of Pittsburgh, USA. Abstract In this topic, three important issues are discussed which concern the theoretical fundamentals and practical operation of a solid oxide fuel cell. The thermodynamic and electrochemical fundamentals of a fuel cell are reviewed in the Section 2. These fundamentals concern the ideal efficiency and energy distribution of a fuel cell’s conversion of chemical energy directly into electrical energy through the oxidation of a fuel. Issues of the chemical equilibrium for a solid oxide fuel cell with internal reforming and shift reactions (in case of methane or natural gas being used as the fuel), are also discussed in detail in this section. The losses of electrical potential in the practical operation of a fuel cell are elucidated in the third section, which includes a discussion about activation polarization, Ohmic loss, and the losses due to mass transport resistance. In the fourth section, the coupled processes of flow, heat/mass transfer, chemical reaction, and electrochemistry, which influence the performance of a fuel cell, are analyzed, and modeling and numerical computation for the fields of flow, temperature, and species concentration, which collectively determine the local and overall electromotive force in a solid oxide fuel cell, are examined in detail. 1 Introduction A fuel cell is a device that converts the chemical energy of a fuel oxidation reaction directly into electricity. It is substantially different from a conventional thermal power plant, where the fuel is oxidized in a combustion process and a thermal- mechanical-electrical energy conversion process is employed. Therefore, unlike heat engines that are subjected to the Carnot cycle efficiency limitation, fuel cells can have energy conversion efficiencies generally higher than that of heat engines [1]. WIT Transactions on State of the Art in Science and Engineering, Vol 10, © 2005 WIT Press www.witpress.com, ISSN 1755-8336 (on-line) doi:10.2495/1-85312-840-6/01 2 Transport Phenomena in Fuel Cells Figure 1: Principle of operation of a SOFC. Ideally, the Gibbs free energy change of fuel oxidation is directly converted into electricity [1, 2] in a fuel cell. As is common in many kinds of fuel cells, the core component of a solid oxide fuel cell (SOFC) is a thin gas-tight ion conducting electrolyte layer sandwiched by a porous anode and cathode, as shown in Fig. 1. For a SOFC, this electrolyte is a solid oxide material that only allows the passage of charge-carrying oxide ions. To produce useful electrical work, free electrons released in the oxidation of a fuel at the anode must travel to the cathode through an external load/circuit. Therefore, the electrolyte must conduct ions while preventing electrons released at the anode from returning back to the cathode by the same route. The oxide ions are driven across the electrolyte by the chemical potential difference on the two sides of the electrolyte, which is due to the oxidation of fuel at the anode. This difference in the chemical potential is proportional to the electromotive force across the electrolyte, which, therefore, sets up a terminal voltage across the external load/circuit. The solid oxide electrolyte has sufficient ion conductivity only at high tempera- tures (from 600–1000 ◦C). The high operating temperature of a SOFC also ensures rapid fuel-side reaction kinetics without requiring an expensive catalyst. In addi- tion, the high temperature exhaust from a SOFC can be directed to a gas turbine (GT); thus, using a SOFC-GT hybrid system, one can achieve an efficiency of at least 66.3% based on the lower heating value (LHV, which means that the electro- chemical product, water, is in a gaseous state) of the SOFC [3–6]. Since it operates via transport of oxide ions rather than that of fuel-derived ions, in principle, a SOFC can be used to oxidize a number of gaseous fuels. In particular, a SOFC can consume CO as well as hydrogen as its fuel, and therefore can be fueled with reformer gas containing a mix of CO and H2 [7, 8]. Recently, ammonia has also been reported as a fuel for SOFCs [9]. Since a SOFC operates under high temperatures, its energy conversion efficiency and component safety are both of concern to industry. In the following sections, the issues to be discussed will include: (1) the thermodynamic and electrochemi- cal fundamentals of the energy conversion and species variation, (2) the potential losses in practical operation, (3) the influence of fluid flow and heat and mass transfer on operational efficiency and safety, and (4) the creation of a numerical model to simulate the performance and the fields of flow, temperature, and species concentration. WIT Transactions on State of the Art in Science and Engineering, Vol 10, © 2005 WIT Press www.witpress.com, ISSN 1755-8336 (on-line) Multiple transport processes in solid oxide fuel cells 3 2 Thermodynamic and electrochemical fundamentals for solid oxide fuel cells To study the energy conversion efficiency and distribution of the conversion pro- cesses in a fuel cell, one must understand the basic principles.The chemical potential and, thereof, electromotive force across the electrolyte involve the interrelation of thermodynamics, electrochemistry, ion/electron conduction, and heat/mass trans- fer. In this section, the fundamentals of thermodynamics and the electrochemistry for a solid oxide fuel cell system are reviewed. The isothermal oxidation of a fuel A with oxidant B can be expressed by the following equation: aA + bB +···→xX + yY +···. (1) The systematic changes of enthalpy, Gibbs free energy, and entropy production in the reaction are related by H = TS + G. (2) In a solid oxide fuel cell, the operating temperature is from 600 ◦C to 1000 ◦C and the pressure of gases is relatively not high. Thus, the gas species of reactants and products can be treated as ideal gases, which allows the chemical enthalpy change to be expressed as: H = (xhX + yhY +···) − (ahA + bhB +···), (3) where the h is the specific enthalpy. When a gas is pure, ideal, and at 1 atm, it is said to be in its standard state. The standard state is designated by writing a superscript 0 after the symbol of interest [10]. The Gibbs free energy which pertains to one mole of a chemical species is called the chemical potential. For an ideal gas at temperature of T and pressure of p, the chemical potential is expressed as: p g = g0 + RT ln , (4) p0 where R is the gas constant and p0 is the standard pressure of 1 atm. One may omit the p0 in the denominator of the logarithm in eqn (4), but in such a case, the pressure p must be measured in atm. The systematic change of the Gibbs free energy in eqn (1) can be expressed in terms of the standard state Gibbs free energy and the partial pressures of the reactants and products: G = (xgX + ygY +···) − (agA + bgB +···) (p /p0)x(p /p0)y ··· = [xg0 + yg0 +···] − [ag0 + bg0 +···] + RT ln X Y X Y A B 0 a 0 b (pA/p ) (pB/p ) ··· (p /p0)x(p /p0)y ··· = G0 + RT ln X Y , (5) 0 a 0 b (pA/p ) (pB/p ) ··· WIT Transactions on State of the Art in Science and Engineering, Vol 10, © 2005 WIT Press www.witpress.com, ISSN 1755-8336 (on-line) 4 Transport Phenomena in Fuel Cells where 0 = 0 + 0 +··· − 0 + 0 +··· G (xgX ygY ) (agA bgB ), (6) which is the Gibbs free energy change of the standard reaction at temperature T (i.e., with each reactant supplied and each product removed at the standard atmospheric pressure, p0 = 1 atm). The theoretical electromotive force (EMF) induced from the chemical potential (G) is the Nernst potential: −G −G0 RT (p /p0)a(p /p0)b ··· E = = + A B ln 0 x 0 y , (7) neF neF neF (pX /p ) (pY /p ) ··· where F(=96486.7 C/mol) is Faraday’s constant. The first part of the right- hand side of the standard reaction is also called the ideal potential, which is denoted by: −G0 E0 = , (8) neF where ne is the number of electrons derived from a molecules of the fuel, when the fuel is oxidized in the reaction of eqn (1). While the Gibbs free energy change, −G, converts to electrical power, the entropy production, −TS, is the thermal energy that is released in the electrochemical oxidation of the fuel. Both the h and g0 are solely functions of temperature for ideal gases, which are given in Tables 1(a) and 1(b) for the gas species involved in the reactions of a SOFC. While the electromotive force in a fuel cell is determinable from the chemical potentials as discussed above, the current to be withdrawn from a fuel cell, denoted by I, is directly related to the molar consumption rate of fuel and oxidant through the following expressions: I I mfuel = ; mO = , (9) fuel 2 O2 ne F ne F O2 where ne is for oxygen, and is the number of electrons per b molecules of oxygen fuel obtained in the electrochemical reaction (in eqn (1)), and ne is the number of electrons derived per a molecules of the fuel. 2.1 Operation with hydrogen fuel If a SOFC operates on hydrogen gas, the oxidation of hydrogen is the only electro- chemical reaction in the fuel cell, which may be expressed by the following chemical equation: 1 H + O = H O (gas). (10) 2 2 2 2 WIT Transactions on State of the Art in Science and Engineering, Vol 10, © 2005 WIT Press www.witpress.com, ISSN 1755-8336 (on-line) Multiple transport processes in solid oxide fuel cells 5 Table 1(a): Enthalpy and standard state Gibbs free energy of species.
Details
-
File Typepdf
-
Upload Time-
-
Content LanguagesEnglish
-
Upload UserAnonymous/Not logged-in
-
File Pages41 Page
-
File Size-