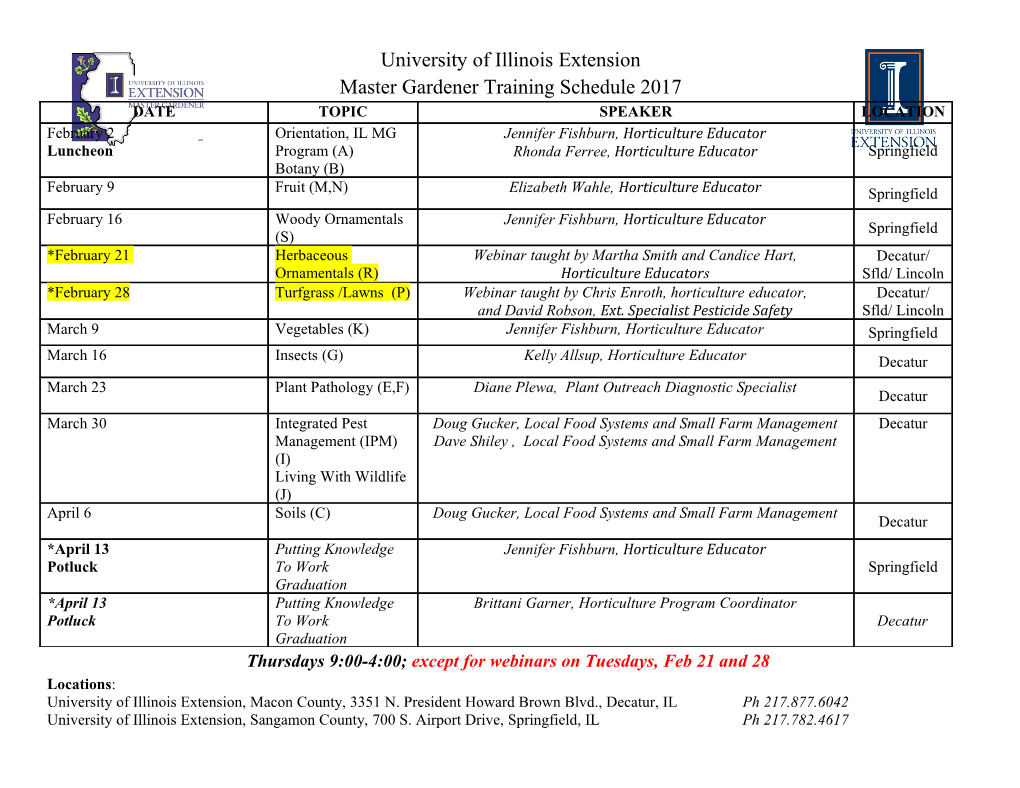
Motility in a Test Tube By Ronald Vale A Key Experiment produced by The Explorer’s Guide to Biology 2 Th e Explorer’s Guide to Biology https://explorebiology.org/ Motility in a Test Tube Ronald Vale, University of California, San Francisco Ronald (Ron) Vale Ronald (Ron) Vale is a professor at the University of California, San Francisco and an investigator with the Howard Hughes Medical Institute. Ron received his Ph.D. in Neuroscience from Stanford University in 1985. His honors include the Lasker Award in Basic Medical Research, the Shaw Prize in Life Sciences, and the Canada Gairdner Award; he was elected to the US National Academy of Sciences and the National Academy of Medicine. Ron believes in the free dissemi- nation of scientifi c knowledge throughout the world. He is the founder of iBiology, a non-profi t organization that produces free videos of talks by leading scientists, and the founder and executive producer of The Explorer’s Guide to Biology. Published on September, 2019 3 Motility in a Test Tube What’s the Big Deal? Cities bustle with the motion of people and commercial goods. Just as goods must be transported from one point to another within a city, molecules also need to be transported within cells to the des- tinations where they are needed. Accordingly, cells have developed roadways (cytoskeletal filaments), engines (protein motors), and cargo containers (membrane organelles) to physically transport mole- cules. These biological transport systems are essential for all cells, but they play especially important roles in neurons, which must transport molecules over very long distances. A failure of the biological transport system can lead to diseases of the nervous system as well as other organs. A cell is about a billion times smaller than a city. How can one study such a miniaturized transport system? Powerful microscopes can be used to visualize small subcellular objects, and the videos in this “Key Experiment” provide a peek at the busy highways inside a nerve cell. In addition, this experiment uses biochemistry to re-build a cell’s transportation system in a test tube. These simplified methods for studying motility led to the discovery of a new motor protein (kinesin) and became powerful tools for understanding how biological motility works. This “Key Experiment” also explains “assays” and “controls” (core concepts in biology) and reveals how unexpected twists and turns can shape research projects and careers. Learning Overview Big Concepts Cells contain thousands of proteins performing many processes. Biochemists try to reduce the complexity in understanding these processes by studying a biological activity outside of the cell (in vitro) and with a minimal number of components. In this “Key Experiment,” the complex process of membrane organelle transport in a nerve cell was rebuilt in vitro from its molecular parts, pro- viding new insights and tools for understanding the basis of biological motility. Terms and Concepts Used Electron microscope (EM), enzyme, gel electrophoresis, fractionation, light microscope, lipid, mem- branes, mitochondria, model organism, neuron, neuron cell body, nucleus, plasma membrane, pro- tein, protein domain, protein purification, sucrose, stem cell Terms and Concepts Explained Actin, adenosine triphosphate (ATP), assay, ATPases, axon, axoplasmic transport, buffer, cyto- skeleton, gel electrophoresis, kinesin, in vitro reconstitution, microtubule, motor neuron, motor pro- tein, organelles, ultracentrifuge, variable Key Experiment Motility in a Test Tube 4 Motility in a Test Tube Introduction Neurons have long, narrow tubes called axons that connect the cell body to the nerve terminal. Membrane organelles move within the axons to transport goods between the cell body and nerve terminal through a process called axonal transport. At the time of this experiment (1984), the mechanism of axonal transport was not known. What Events Preceded the Experiment? It is important to pick a good organism to study a biological problem. For studying axonal trans- port, squid was a good choice because it has a “giant axon” that can be easily dissected out of the squid. In the early 1980s, Allen, Brady, and Lasek observed organelles moving inside of the axon using a video microscope, one of the first occasions in which video cameras and computers were used to record images from a microscope rather than just looking with the naked eye. The cytoskeleton is a network of protein filaments inside of cells. Two main cytoskeletal systems found in all eukaryotic cells are actin filaments and microtubules. Electron microscopy studies revealed that microtubules serve as tracks for long-distance transport of organelles in axons. Setting up the Experiment To learn more about how axonal transport works, the investigators isolated the components that they thought were needed for transport and then recombined them in a test tube. They then used a microscope to examine the combined mixture to observe if organelles were transported along microtubules. This type of experiment is called in vitro reconstitution (taking a system apart and putting it back together in a test tube). The isolated components were: A) Microtubules (the tracks for movement) made from a purified protein called tubulin. B) Adenosine triphosphate (ATP; the chemical energy source). C) Membrane organelles (the cargo) from the giant axon, which were isolated by the method of sucrose density gradient ultracentrifugation. An ultracentrifuge spins samples at high speeds, allowing biological materials to be separated on the basis of both size and density. D) Soluble protein fraction (free proteins that are neither associated with organelles nor the cytoskeleton) were also isolated from the giant axon. Doing the Key Experiment The in vitro reconstitution experiment involved mixing various combinations of the components described above and then looking through a microscope to observe if the isolated organelles would move along the microtubule tracks. 5 Motility in a Test Tube The scientists first combined 1) microtubules, 2) membrane organelles, and 3) ATP. They thought that this combination might work as it was expected that the motors are bound to organelles. However, no movement was observed. Then, they thought that a component might be missing and hence added the soluble protein fraction. Now the organelles started moving along microtubules, suggesting that this fraction contained a key ingredient needed for the movement. The key ingredient in the soluble protein fraction proved to be a motor protein itself. When they combined microtubules, the soluble protein fraction, and ATP (but no organelles), surprisingly, they observed microtubules moving over the surface of a glass microscope slide. A follow-up experiment showed that the motor protein stuck onto the glass and the glass-bound motor pro- teins propelled the microtubules along the surface. Small plastic beads also could bind to the unknown motor protein from the soluble protein frac- tion. The motor protein would transport the beads along the microtubules in a manner that looked similar to the transport of membrane organelles inside of axons. This experiment demonstrated that axonal transport could be reconstituted from isolated compo- nents. It also led to new experimental strategies (called “assays”) for studying motor proteins— the movement of microtubules across a glass surface and the movement of plastic beads along stationary microtubules. These new assays, which did not involve membrane organelles (complex objects themselves), provided new and simple ways of measuring the activity of motor proteins. What Happened Next? The simplified in vitro motility assays provided means to isolate a new motor protein from the axon, which was named “kinesin.” In addition to helping in the identification of a new motor protein, these assays provided powerful tools for understanding how motor proteins convert chemical energy from ATP into movement. Closing Thoughts Serendipity plays a big role in scientific research. The in vitro motility assays of microtubules mov- ing across a glass surface and plastic beads moving along stationary microtubules were unantic- ipated at the start of the experiment and proved to be the biggest advancement. Guided Paper Vale, R.D., Schnapp, B.J., Reese, T.S., and Sheetz, M.P. Organelle, bead and microtubule translocations pro- moted by soluble factors from the squid giant axon. Cell 1985;40:559–569. 6 Motility in a Test Tube Introduction Could a single evening in the laboratory change the course of one’s life? This is the story of such an evening. I was a 25-year-old student in a combined PhD/MD training program at Stanford University. At the time of this story, I was wrapping up the research for my PhD degree and, in 3 weeks, was scheduled to stop my research and transition to the final phase of my MD training. I had already completed enough work to obtain my PhD. But I wanted to end my research work with a bang and not a whimper. But I was not getting any results. Were the experiments too difficult? Or was I just clumsy? As my experiments were not going well, it was tempting to give up. However, my fascination with the research kept me going. Lying in front of me was a great puzzle that I wanted to solve. The clock was ticking; my scientific research would come to halt for at least 2 years after I returned to medical school. It was now or never. I was studying how neurons transport materials (proteins and membranes) from the location where they are made to the destinations where they are needed. Most biomolecules are made in the cell body, where the nucleus is located. However, some of these molecules have to be shipped to the nerve terminal. Although all cells need to transport materials, the distance between the cell body and nerve terminal can be much greater than the distance across most other cells in the body. A motor neuron, for example, has its cell body in the spinal cord and its nerve terminal could be located at a muscle in your foot, a distance of around 1 m (Figure 1).
Details
-
File Typepdf
-
Upload Time-
-
Content LanguagesEnglish
-
Upload UserAnonymous/Not logged-in
-
File Pages32 Page
-
File Size-