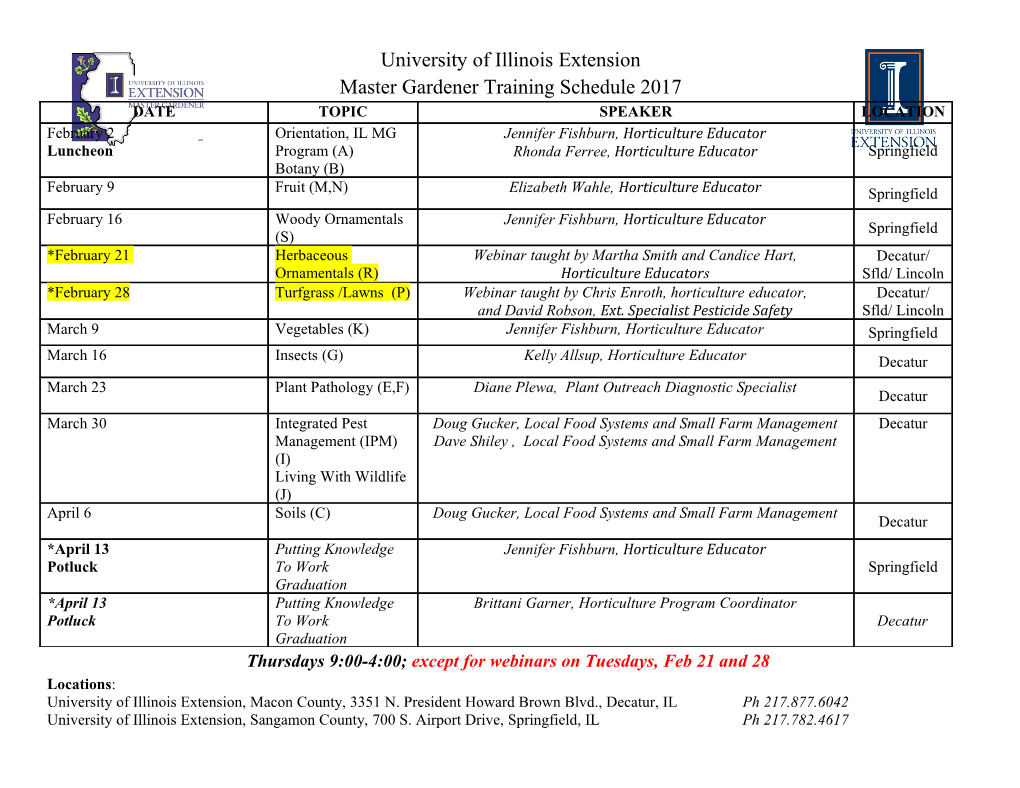
Evolutionary Conservation of Annexins in Petromyzon marinus, Sea Lamprey, a Neurodegenerative Disease Model Caitlin Quaempts Abstract Annexin proteins are a family of well characterized calcium-dependent membrane binding proteins with highly conserved sequences, but still a diversity of function and localization. There are 12 annexins observed in humans, with annexins A2, A5, A6, and A7 having extensive research in their roles in endocytosis, exocytosis, and reducing toxic effects of aggregated proteins associated with neurodegenerative diseases. Due to the identification of multiple annexins playing roles in neuronal processes, the need to further examine the remaining annexin proteins in a neuronal environment may provide vital information in their pathophysiological roles in neurodegenerative diseases. Lamprey provide large axons for observation of this interaction and their conserved neuronal pathways with humans will allow for application of the findings, making them a good model organism. Genome, transcriptome, and phylogenetic tree analysis were performed to identify annexins present in lamprey. Annexins A2, A4, A7, A8, A10, A11, and A13 were identified in lamprey with duplication events in A2, A7, and A13 which supports previous findings. Unidentified annexins were also present in lamprey and were named annexins A14, and A15. These lamprey annexin proteins should be validated with a more complete taxonomical phylogenetic tree and can then be applied to the model organism to observe specific neuronal roles of individual annexins. Introduction: Annexin proteins are known as a family of calcium-dependent membrane binding proteins consisting of 12 subfamilies in mammals that may differ for other vertebrates, consisting of the Annexin A proteins (Moss & Morgan, 2004). There are also annexin B, C, D, and E 1 proteins for invertebrates, fungi/unicellular eukaryotes, plants, and protists, respectively, (Moss & Morgan, 2004). The fact that these proteins conserved throughout all eukaryotes and are able to be identified as annexins, even within the protists, indicates a retained function of this protein that plays a fundamental role in eukaryotic cell biology (Moss & Morgan, 2004). The ancestral founder of vertebrate annexins have been found to be ANXA13, ANXA7, and ANXA11, by multiple sources (Moss & Morgan, 2004; Morgan et al., 2004; Morgan et al., 2006). The core domain of the protein is widely conserved among all 12 subfamilies (Morgan, et al., 2004). This core domain consists of four similar repeats of about 70 amino acids in length each, which form 5-α helices per domain (Morgan, et al., 2004). These domains are characteristic of a “type 2” calcium binding motif, which has a sequence of GxGT-38 residues-D/E (Moss & Morgan, 2004). While the core amino acid sequence is largely conserved, the N-terminus and cytosolic exposed amino acids show an increased chance of sequence variety which determines protein and membrane binding interactions (Moss & Morgan, 2004). This in addition to the posttranslational modifications, like phosphorylation, can provide an explanation for the differentiation of localization and function of annexins. The different annexins have been found to interact with different proteins, such as ANXA1 with epithelial growth factor receptor, a receptor that controls proliferation, apoptosis, and angiogenesis, that is upregulated in many cancer types, ANXA2 can interact with tissue plasminogen activator, which contributes to breakdown of clots, and additional protein-protein interactions (Moss & Morgan, 2004). Although the core domain is conserved, it is clear there is a wide variation of functionality between the individual annexin proteins. Each individual annexin gene is not expressed in every cell in the body. Annexins A1, A2, A4, A5, A6, A7, and A11 have been found to be ubiquitously expressed, but other protein 2 members are more selective within the body. In addition to localization at the presynaptic terminal, some annexins may localize to the cell surface, like ANXA1, or to the nucleus at times, such as ANXA2 and ANXA11 (Moss & Morgan, 2004). Annexins have been found to function in calcium regulated exocytosis, endocytosis, and stabilization of organelles and the plasma membrane (Moss & Morgan, 2004). Specifically, annexin A7 has been found to play a role in vesicle trafficking due to its role in calcium signaling (Rescher & Gerke, 2004). Additionally, ANXA7 mediates the calcium-dependent hydrolysis of GTP that initiates exocytotic membrane fusion in neurons and chromaffin cells (Caohuy, Srivastava & Pollard, 1996). For endocytosis, cell surface expression of ANXA6 could possibly have an important role in regulating the budding of clathrin-coated vesicles (Grewal et al., 2000). In addition to ANXA6, ANXA2 has also been investigated in respect to its role in endocytic processes. This was performed by using RNAi to downregulate express of ANXA2 in HeLa cells. Results showed defects in endosomal carrier vesicles, specifically with its shape and redistribution of recycled the vesicles (Rescher & Gerke, 2004). In terms of pathological diseases, ANXA5 has even been identified as a marker in neurodegenerative diseases, such as Alzheimer’s disease, Dementia with Lewy bodies, and Parkinson’s Disease, that have overexpression of toxic protein aggregates like α-synuclein (Sohma et al., 2013). It has been hypothesized that ANXA5 is upregulated in response to protecting the neuron from calcium-induced damage (Sohma et al., 2013). ANXA5 has also been found to interact with the toxic proteins, such as α-synuclein inclusions, and eventually reduce the toxic effect of these (Bedrood et al., 2009). It appears ANXA2/ANXA6, ANXA7, and ANXA5 play a role in endocytosis, exocytosis, and reduces the toxic effects of proteins associated with neurodegenerative diseases, respectively. Neurodegenerative diseases, such as Alzheimer’s Disease and Parkinson’s Disease 3 (PD), also exhibit defects along the endocytic and exocytotic pathways in addition to the toxic effects of proteins like α-synuclein. Because the ANXA2, ANXA6, ANXA5, and ANXA7 play a role in each of these pathways, further studies of annexins could contribute to further understanding of the pathophysiology of neurodegenerative diseases. Parkinson’s Disease (PD), the second most common neurodegenerative disorder of the brain, is characterized by a loss of the dopaminergic neurons in the substantia nigra pars compacta region of the brain (Vallone et al., 2000). This part of the brain is responsible for voluntary movement, causing motor abnormalities such as stiffness, bradykinesia and resting tremor (Chinta & Anderson, 2005). The exact cause of PD is still unknown, and there are no currently available treatments that have an effect on the disease or its progression. Increased understanding of the cellular mechanisms involved, such as the regulatory role of annexins within neurons, and their evolutionary conservation may guide the identification of novel targets for treatments. In order to investigate the proteins involved in the degeneration of these neurons, the normal functions of neurons must be understood. Neurons use endo and exocytosis to transmit chemical signals, known as neurotransmitters, between one another. When this pathway is altered or defective, it can possibly lead to cell death. These neurotransmitters are packaged in organelles, known as synaptic vesicles, that are composed of a phospholipid membrane (Lodish et al., 2000). When the neuron receives an influx of calcium ions, these synaptic vesicles fuse to the plasma membrane in a process known as exocytosis, and release their neurotransmitters to the receiving neuron in order to propagate the signal (Augustine et al., 1999). After exocytosis, there is a need to regenerate the synaptic vesicle that was just lost through a process called endocytosis. This occurs through a number of regulated pathways, such as clathrin-mediated endocytosis, which causes the plasma membrane to invaginate and recreate another synaptic 4 vesicle in order to complete this recycling pathway (Augustine et al., 1999). Recycling of synaptic vesicles is a highly regulated process, so it is possible that when one regulatory protein is present at irregular levels, it can lead to defects in neuronal transmission. Studies such as Busch et al., (2014), and Banks et al (2020), have investigated the defects seen in neurons that mimic conditions of neurodegenerative diseases. This study investigated what happens in neurons immediately after injecting axons with α-synuclein, the protein seen in excess in PD patients. This showed a decrease in synaptic vesicles and irregular invaginations of the plasma membrane in comparison to control samples, indicating endocytic and exocytic defects in this synaptic vesicle recycling pathway in response to excess α-synuclein (Busch et al., 2014; Banks et al., 2020). Excess α-synuclein also interrupts the formation of the SNARE complex, a protein that regulates endocytosis, therefore inhibiting the fusion of synaptic vesicles to the plasma membrane (Bridi & Hirth, 2018). Additionally, α-synuclein is found to disrupt cellular inorganic ion homeostasis like calcium, that leads to cell death (Soma et al., 2013). It is possible that α-synuclein interacts with other regulatory proteins, like annexins, in a stoichiometrically specific interaction. Therefore, when α-synuclein is in excess, this interaction is altered and causes the synaptic vesicle recycling defects observed. Annexins play a role in
Details
-
File Typepdf
-
Upload Time-
-
Content LanguagesEnglish
-
Upload UserAnonymous/Not logged-in
-
File Pages31 Page
-
File Size-