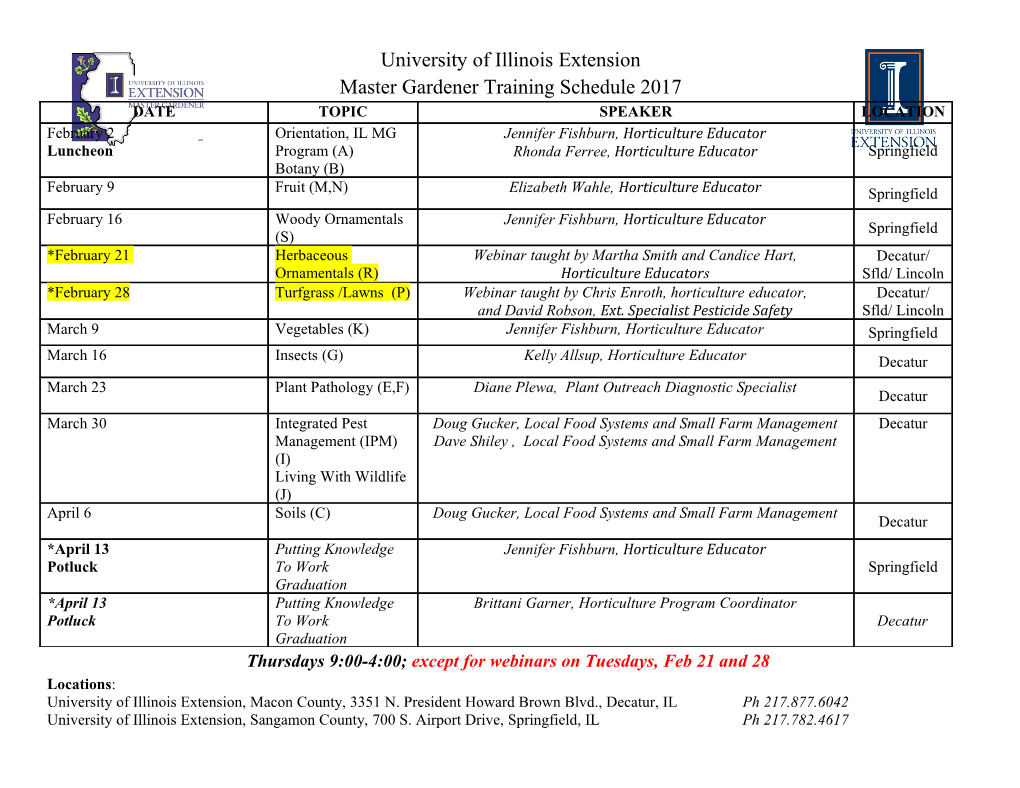
Lecture #1 Today’s lecture • Pitot-static systems ASE 167M Lecture One • Bernoulli’s equation • Airspeed definitions • Flight Instruments Aircraft Systems • Flight control systems Flight 1 Briefing • Flight 1 briefing Revised by Greg Holt 2/2/2004 ASE167M Lecture 1 2 Lecture #1 Lecture #1 Pitot-Static Systems Pitot-Static Systems (2) • Essential requirements in the measurement of a/c performance are: (1) state of atmosphere and (2) relative motion between the a/c and the air mass ⇒ air data systems • Of the primary flight instruments (Altimeter, airspeed, VVI, compass, turn coordinator), the first three use the Pitot-static system • The Pitot-static systems, drive instruments (movement of a pointer mechanically) that use difference between static and total pressure, or just static pressure (independent of electrical power supply) • System includes a pitot tube for measuring impact (ram) pressure, and static ports for measuring barometric static pressure. 2/2/2004 ASE167M Lecture 1 3 2/2/2004 ASE167M Lecture 1 4 Lecture #1 Lecture #1 Bernoulli’s Equation Airspeed Definitions • Total pressure at a given point is that which would exist if • True Airspeed: Solve (1) for V: the flow were slowed down isentropically to zero. ()− = 2 PT P Vtrue ⇒ Function of 2 variables • Assumptions? ρ V1,P1 Flow Pitot Tube – Isentropic V2=0 ρ = ρ (Sea LevelStandard Atmosphere) – Incompressible P2Static Port –Keep sl – P and ρ are corrected for at flight condition P P T • Calibrated Airspeed: • Derived from Newton’s 2nd Law (F = ma) – Speed read on a perfect airspeed indicator (i.e. no instrument error PP21−=1 ρ V2 2 1 and true static pressure) 1 – Corrections may be particular to a/c and instruments P+==ρ V2 P const. along streamline ()1 2 T – Take into account position errors =+∆+∆ Static Dynamic Total VVcalibrated indicated VV posit.. inst Pressure Pressure Pressure 2/2/2004 ASE167M Lecture 1 5 2/2/2004 ASE167M Lecture 1 6 Lecture #1 Lecture #1 Airspeed Definitions (2) Airspeed Definitions (3) • white arc γ −1 • Equivalent Airspeed 2γ p pp− γ ⇒ V =+− 110 true – flap operating range – Corrected for compressibility effects γρ−1 p – stall speed (with full flaps + landing (1.4)γγratio of specific heats⇒ = for air – Corrected for altitude/density changes gear – landing configuration) to – For incompressible flow we use max. flap operating speed 2()PP− ρ ρ qV==11ρρ2 VV2 ⇒ ==T V • green arc 22sl equiv calρρ true slsl – normal operating range = – stall speed (power-off in clean – We will assume: VVequiv cal configuration) to caution range • yellow arc • Indicated Airspeed – caution range – As measured by airspeed indicator •red line – We will assume: = – never exceed speed Vindicated Vtrue 2/2/2004 ASE167M Lecture 1 7 2/2/2004 ASE167M Lecture 1 8 Lecture #1 Lecture #1 Altimeter Vertical Velocity Indicator (VVI) • Measures the weight of the air above the A/C ⇒ fly at a constant pressure • Rate of static pressure level change is measured to give −β Rg/ 0 Tp0 an indication of rate of H =− 1 β p 00 altitude change. • Pressure typically decreases, in the lower atmosphere, by a known amount with altitude. • System exhibits a 4-8 second (standard lapse rate) delay before steady state ≈ - 1.00”Hg/1000 ft reading is accurate. • Static pressure readings are compared • Shows Trend (immediate to a table of pressures to calculate indication) and rate altitude (stabilized) of climb 2/2/2004 ASE167M Lecture 1 9 2/2/2004 ASE167M Lecture 1 10 Lecture #1 Lecture #1 Example Problem Example Solution (1) • Altimeter and airspeed indicator read 8000 ft. • True Airspeed and 120 mph, respectively. Assume that all instruments are perfect. ρ = slug SL 0.00237 3 • A pitot-static tube measures the dynamic ft pressure. ρ = slug 8000 ft 0.00186 3 – What is the true airspeed? ft slug • Assume the a/c flies at the same altitude and 0.00237 ρ ft 3 enters an area of lower pressure. V = V SL =120mph true cal ρ slug – What happens to the altimeter reading? 0.00186 ft 3 – What happens to the airspeed indicator reading? V =135.4mph – What happens to the VVI reading? true 2/2/2004 ASE167M Lecture 1 11 2/2/2004 ASE167M Lecture 1 12 Lecture #1 Lecture #1 Example Solution (2) Gyroscopic Systems • (altitude) As the altitude increases, static • Attitude indicator, heading indicator, and turn & pressure decreases. Therefore, the altimeter will slip indicator or turn coordinator. show an increase. • These devices exploit two properties: (1) rigidity • (airspeed) Assuming constant density, there will be no change in the airspeed indication as the and (2) precession which are based upon the dynamic pressure measured by the pitot-static conservation of angular momentum. tube will not change. – Rigidity is the property which resists any force • (VVI) The static pressure will appear to have tending to change the plane of rotation of the rotor. decreased with time; therefore, the VVI will show – Precession is the actual change in the direction of the a climbing rate. plane of the rotation under the influence of an applied force. 2/2/2004 ASE167M Lecture 1 13 2/2/2004 ASE167M Lecture 1 14 Lecture #1 Lecture #1 Gyroscopic Systems (2) Attitude Indicators • Universally mounted gyroscope (rotates free in any plane) • Horizon bar is attached to a gyro to remain parallel to natural horizon (Gyro acts as a pendulum) 2/2/2004 ASE167M Lecture 1 15 2/2/2004 ASE167M Lecture 1 16 Lecture #1 Lecture #1 Attitude Indicator (2) Heading Indicators • Formerly called the Directional Gyro • Heading indicator is mounted vertically – Susceptible to precession errors 2/2/2004 ASE167M Lecture 1 17 2/2/2004 ASE167M Lecture 1 18 Lecture #1 Lecture #1 Turn Coordinator or Turn-and-Slip Flight Control Systems Indicator • Displays rate and quality of turn • Elevators • Turn rate, bank angle, and velocity related when – Horizontal surfaces on tail of a/c that induce pitch performing a coordinated turn • Ailerons • “Step on the ball” – Horizontal surfaces on wing of a/c that act in opposite directions to induce roll • Rudder – Vertical surface on tail of a/c that induces yaw • Trim Tabs – Supplemental surface on elevators, ailerons, and rudder than can be used to create “fingertip” yoke requirement under continual control surface displacement 2/2/2004 ASE167M Lecture 1 19 2/2/2004 ASE167M Lecture 1 20 Lecture #1 Lecture #1 Flight Control Systems (2) Flight Control Systems (3) • Flaps – increase lift – increase drag Flaps 2/2/2004 ASE167M Lecture 1 21 2/2/2004 ASE167M Lecture 1 22 Lecture #1 Lecture #1 Power Plant Systems (1) Power Plant Systems (2) • Carburetor : Use Carburetor Heat • Magnetos Test L and R 2/2/2004 ASE167M Lecture 1 23 2/2/2004 ASE167M Lecture 1 24 Lecture #1 Lecture #1 Power Plant Systems (3) Power Plant Systems (4) Manifold Pressure (MP) RPM Control Fuel Flow •Cowl Flaps • Propellers or Throttle Control Control – RPM controls Pitch of the propellers RPM Control Manifold Pressure Fuel Flow Control (MP) or Throttle Control 2/2/2004 ASE167M Lecture 1 25 2/2/2004 ASE167M Lecture 1 26 Lecture #1 Lecture #1 Lab Schedule Flight 1 Briefing • Orientation Flight: Expand cockpit familiarity and develop the basic concepts for a/c performance • Get the best rate-of-climb and best angle-of-climb and its respective velocities (Vx and Vy) 2/2/2004 ASE167M Lecture 1 27 2/2/2004 ASE167M Lecture 1 28 Lecture #1 Lecture #1 Equations of Motion in a Vertical Flight 1 Briefing (2) Plane • In theory we would have to get those •EOM: h L x performances at a constant altitude ⇒ wait until ANGLE OF ATTACK b T α θ flight #5 V x γ w PITCH ANGLE O xh H=const FLIGHT PATH ANGLE D xw – Relative Wind direction W x – x body direction E i b xh – x local horizon direction k x Read R/C at constant altitude xV& = cosγ & = γ hVsin g VT& =+−−cos()ε α DW sinγ • We are going to climb and read R/C for several W 0 g γ =++−()εα γ altitudes, velocities and power settings and we & TLWsin0 cos WV will assume that they were read at H=const. WCT& =− 2/2/2004 ASE167M Lecture 1 29 2/2/2004 ASE167M Lecture 1 30 Lecture #1 Lecture #1 Flight 1 Briefing (2) Flight 1 Briefing (3) Event Rate of Climb Airspeed • Record airspeed MP = Manifold Pressure (“ of Hg) (ft/mim) (knots) •Plot dh/dt vs. V for 5 points in the flight (Steps 3 to 7). Fit data to Preflight Checklist; RPM = Max ; MP = Max 0 0 and rate of climb Takeoff (Rotate at 75 knots); Raise Landing Gear 0 75 2 Climb at 100 knots to 2000 ft MSL; Read R/C at 1800 ft; Set RPM = Max; 100 = = + + data for climbs and MP = Max R / C h& a0 a1V a2V Climb at 120 knots to 3000 ft. Read R/C at 2800 ft 120 descents. Climb at 85 knots to 4000 ft. Read R/C at 3800 ft 85 • Go to specified Climb at 70 knots to 5000 ft. Read R/C at 4800 ft 70 – Use a least squares curve fit (available on most plotting Climb at 65 knots to 6000 ft. Read R/C at 5800 ft 65 packages) airspeed, allow time Level off at 6000 ft. Set autopilot and Stabilize for 1 mim. Read Airspeed 0 Reduce RPM = 2300; MP = 21 “Hg. Read Airspeed after stabilized 0 for VVI to stabilize Reduce RPM = 2000; MP = 20 “Hg. Read Airspeed after stabilized 0 Set RPM = 2400; MP = Max. Read Airspeed after stabilized. 0 dh = γ (to ensure steady • Calculate max h& max and from parabolic constants and Lower ½ flaps.
Details
-
File Typepdf
-
Upload Time-
-
Content LanguagesEnglish
-
Upload UserAnonymous/Not logged-in
-
File Pages10 Page
-
File Size-