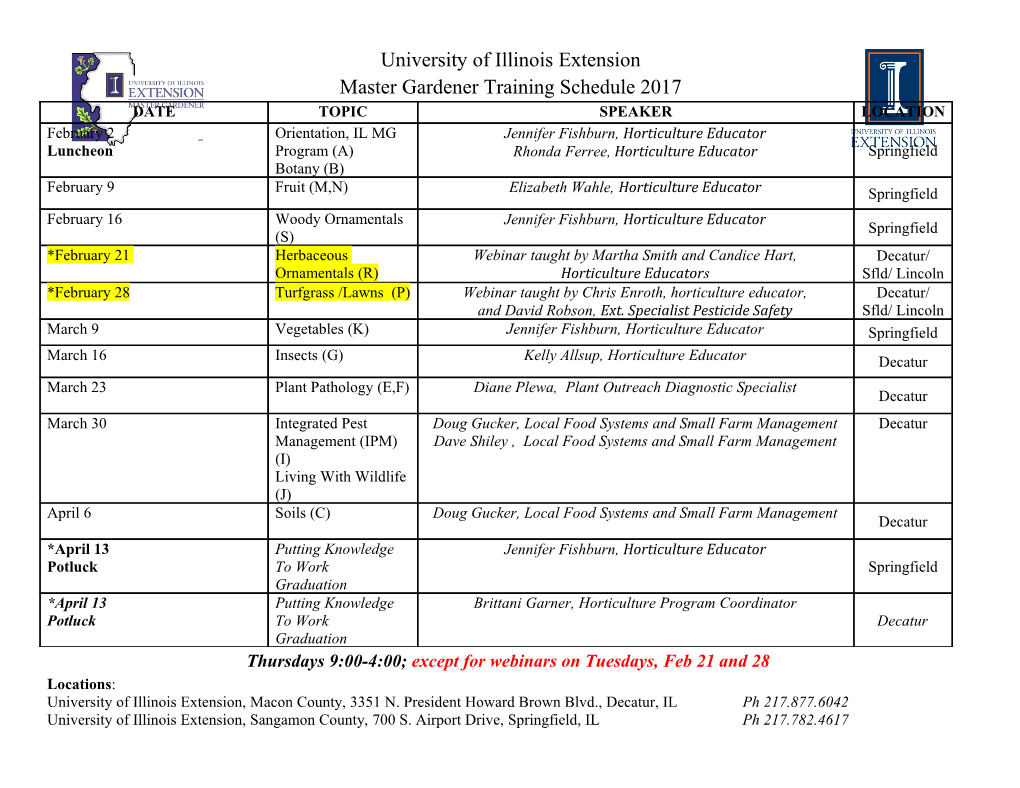
Matrix Assisted Laser Desorption/Ionization Orthogonal Acceleration Time-of-Flight Mass Spectrometry: Development and Characterization of a New Instrument By David Sean Selby A candidate for the degree of Doctor of Philosophy Submitted to the University of New South Wales December 2002 Certificate of Originality I hereby declare that this submission is my own work and to the best of my knowledge it contains no material previously published or written by another person, nor material which to a substantial extent has been accepted for the award of any other degree or diploma at UNSW or any other educational institution, except where due acknowledgment is made in the thesis. Any contribution made to the research by others, with whom I have worked at UNSW or elsewhere, is explicitly acknowledged in the thesis. I also declare that the intellectual content of this thesis is the product of my own work, except to the extent that assistance from others in the project’s design and conception or in style, presentation and linguistic expression is acknowledged. David Selby Abstract The performance of a linear matrix assisted laser desorption/ionization mass spectrometer (MALDI-oa-TOFMS) was improved with more reproducible sample preparation methods, a higher rate digitiser for integrating signals and customisable computer control, data acquisition and analysis in the LabVIEW programming environment. This resulted in a ~20% improvement in resolution (up to 4,400) and enabled measurement of desorption velocities of 1,000 - 1,800 ms-1 for analytes with m/z 615 – 1,350 Da, with matrix ion velocities being 4,000 – 4,800 ms–1. Detector limitations and restrictions on source axis energy (and hence velocity) required for the analysis of ions prevented detection of other species with this instrument. A 20 kV reflecting geometry MALDI-oa-TOFMS was constructed to overcome these limitations and extend the mass range. This mass spectrometer was able to analyse ions desorbed with a wide range of energies (32 – 197 eV). The resolution was found to be 8,000 -10,000. Best mass accuracy was 15-80 ppm (internal standards ). External calibration gave larger mass errors, mostly due to timing jitter, but the mass axis was stable for >2 weeks. Mass accuracy was independent of the analyte and matrix used. Ions with m/z of ~10,000 - 20,000 Da were observable with the use of a pulsed lens in the target region. This lens increased signal approximately 20 times, but degraded resolution. The detection limit of the instrument (sample consumed) was estimated to vary from 10 – 90 fmol, by extrapolation, with more moles required at higher m/z. The microsphere plate (MSP) electron multiplier used in the reflecting instrument was found to have a temporal response of < 1 ns FWHM, but with a low secondary electron conversion efficiency, making it unsuitable for high m/z species. Experiments were also performed with a novel rectangular mesh grid, which (in correct orientation) provided similar resolution to conventional square mesh grids, but with significantly improved transmission and hence sensitivity. i Acknowledgments Before commencing this project I knew little about the practicalities of automated instrument control, design and characterization, while by its completion I had learned enough to become an important member of a team. I would like to thank a number of individuals who contributed to this process. Firstly I would like to thank the two scientists who made this project possible: my supervisor, Associate Professor Michael Guilhaus, who in addition to his thorough supervision often made one feel more like a colleague than a student, for which I especially thank him; my co-supervisor, Dr Victor Mlynski, who was responsible for modifying the linear oa-TOF instrument into MALDI mode and largely responsible for the physical design and construction of the reflecting geometry MALDI-oa-TOF instrument. Secondly I would like to thank others who made a significant contribution to the project: Paul Hallohan and Mitchell Davis from the School of Chemical ScienceÕs Mechanical Workshop, who fabricated parts to our exacting standards; John Morgan of the School of Chemical ScienceÕs Electronics Workshop, who provided assistance on innumerable occasions concerning computers and electronics; Dr Len Cherkson of the School of Chemical ScienceÕs Electronics Workshop, who designed our push out pulse generator; Dr Joseph Brophy, operator of the School of ChemistryÕs Mass Spectrometry Unit, who provided samples and advice; Dr Keith Fischer and Rui Zang, who provided samples; and Associate Professor Mark Duncan formerly of the Ray Williams Biomedical Mass Spectrometry Facility and Martin Bucknall and Ann Poljack of the Ray Williams Biomedical Mass Spectrometry Facility, who provided advice, samples and access to a commercial MALDI instrument. Thirdly I would like to thank those who helped to make the stay enjoyable: My fellow students in the Analytical Mass Spectrometry group, Patricia Calderon, Brett Ireland, Mark Lewin (who was also an excellent proof reader), Nirand Pongpun and Nageeb Sousou, and Dr Robert Goldsack of Tim-Tam fame. Finally (and perhaps most importantly) I would like to thank my parents, Janice Selby and Luc Szulevicz, without whose upbringing I would not have turned out the way I did or have had the persistence to complete this thesis. 179 Contents Chapter 1: Introduction 1.1 Overview of Thesis 1 1.2 Time-of-Flight Mass Spectrometry 2 1.2.1 Introduction to Mass Spectrometry 2 1.2.2 The Time-of-Flight Analyser 3 1.2.3 Resolution and TOF Mass Analyser Performance 5 1.2.4 Other Measures of TOFMS Performance 6 1.2.5 Improving Resolution: Ion Optics, Time-Lag Focusing and the Ion 8 Mirror 1.2.6 Coupling with Continuous Ionization Sources 10 1.3 Orthogonal Acceleration Time-of-Flight Mass Spectrometry 11 1.3.1 Introduction to Orthogonal Acceleration Time-of-Flight Mass 11 Spectrometry 1.3.2 Principles Specific to oa-TOFMS 12 1.3.3 Types of oa-TOFMS Instruments and Applications 15 1.4 Matrix Assisted Laser Desorption/Ionization 18 1.4.1 The Development of MALDI 18 1.4.2 Theory of Desorption/Ionization 20 1.4.3 Performance Limiting Factors: Initial Kinetic Energy, Detector 20 Efficiency and Reproducibility 1.4.4 Improving TOFMS Performance with Sample Preparation 22 1.4.5 Improving Performance with Instrumentation: Ion Mirrors and Delayed 23 Extraction ii 1.4.6 Range of Applications: Types of Analyte and Obtaining Structural 24 Information 1.5 MALDI-oa-TOF Combination 26 1.5.1 MALDI-oa-TOF Instrumentation and Applications 26 1.5.2 MALDI with Hybrid Sector/oa-TOF Analysers 27 1.5.3 Why Combine MALDI with non-tandem oa-TOF Analysis? 29 1.6 Computer Simulations of oa-TOFMS 31 1.6.1 The Importance of Simulations in the Design and Characterization of 31 oa-TOF Instruments 1.6.2 I-Opt 31 1.6.3 SimTOF 33 1.7 Aims 35 Chapter 2: Instrumentation and General Methods 2.1 Overview of Instruments and Methods 36 2.2 Prototype Linear MALDI-oa-TOF 36 2.3 MALDI-oa-TOF with an Ion Mirror 37 2.4 A Commercial Instrument: The Voyager 39 2.5 Sample Preparation 39 2.5.1 Matrices, Analytes and Solvents 40 2.5.2 Cleaning of Sample Slides 40 2.5.3 Dried Droplet Sample Preparation 40 2.5.4 Electrospray Sample Deposition 41 iii Chapter 3: Computerised Instrument Control and Analysis 3.1 A Brief Introduction to LabVIEW and Virtual Instruments 43 3.2 Computer Hardware 44 3.3 Instrument Control 45 3.3.1 Computer Controlled Laser Triggering and oa Pulse 45 3.3.2 Control and Monitoring of Power Supplies 46 3.4 Data Acquisition and Analysis 48 3.4.1 Data Acquisition 48 3.4.2 Analysis 50 3.5 Other Programs and Macros 51 Chapter 4: Improving Performance of the Linear MALDI-oa-TOF 4.1 Importance of Improving Performance 53 4.2 Optimising Dried Droplet Sample Preparation 53 4.2.1 Dried Droplet Sample Preparation Methods 53 4.2.2 Appearance of Target and Spectral Quality for Dried Droplet Samples 54 4.3 Electrospray Deposition Sample Preparation 55 4.3.1 Electrospray Deposition Procedures 55 4.3.2 Appearance of Target and Quality of Spectra Obtained with 56 Electrospray Deposition 4.4 Using a Faster Digitiser and the Effects of Laser Power 58 4.4.1 Procedures Used in Assessing Effects of Digitiser and Laser Power 58 4.4.2 Measured Effect of Digitiser Rate 59 4.4.3 Effect of Laser Power 60 4.5 Summary of Early Experiments and Sample Preparation 61 iv Chapter 5: Fundamental Studies – Desorption Velocity/Energy Experiments on the Linear Instrument 5.1 Importance of Desorption Velocity/Energy 62 5.2 Velocity Distribution in the Desorption Axis 63 5.2.1 Introduction: Published MALDI Desorption Velocities and Relevance to 63 oa-TOF Instrument Geometry 5.2.2 Experimental: Methods used to Measure Source Axis Velocity 65 Distribution 5.2.3 Results and Discussion: Source Axis Velocity and its Effect on 66 Instrument Performance 5.3 Correlation of Velocity and Position in the TOF Axis 67 5.3.1 Relevance of Correlation or Lack of Correlation of Position and 67 Velocity to Instrument Performance 5.3.2 Experiments and Simulations used to Determine the Degree of 68 Correlation 5.3.3 Comparison of Experimental Results with Simulations 70 5.4 Extension to Other Samples: Higher m/z and Matrix Effects 71 5.4.1 Methods of Sample Preparation and Analysis 72 5.4.2 Analysis of High m/z and HCCA Matrix Results 73 5.4.3 Conclusions – Limits of the Instrument 75 Chapter 6:Testing and Improving MALDI-oa-TOF with an Ion Mirror 6.1 Introduction 76 6.2 Initial Experiments with Fullerene Standard, Gramicidin S and Insulin in 77 DHB Matrix
Details
-
File Typepdf
-
Upload Time-
-
Content LanguagesEnglish
-
Upload UserAnonymous/Not logged-in
-
File Pages299 Page
-
File Size-