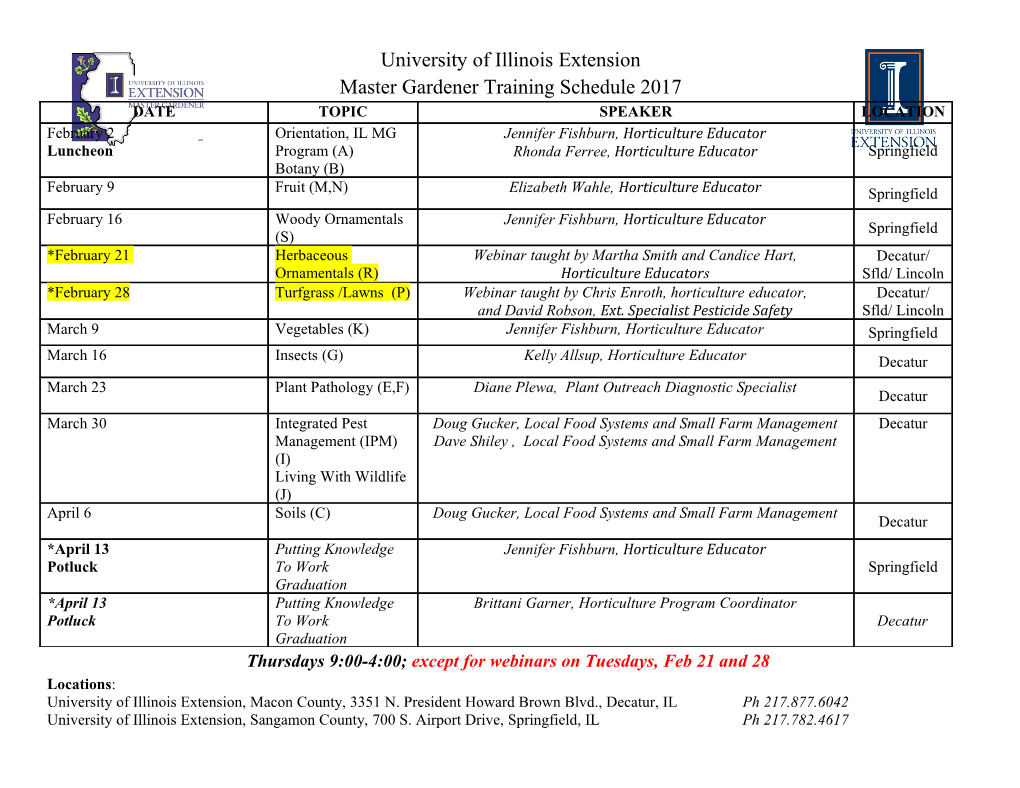
A&A 567, A105 (2014) Astronomy DOI: 10.1051/0004-6361/201423971 & c ESO 2014 Astrophysics The colour–magnitude relation of globular clusters in Centaurus and Hydra Constraints on star cluster self-enrichment with a link to massive Milky Way globular clusters J. Fensch12,S.Mieske2, J. Müller-Seidlitz3 , and M. Hilker3 1 École polytechnique, route de Saclay, 91128 Palaiseau, France e-mail: [email protected] 2 European Southern Observatory, Alonso de Cordova 3107, Vitacura, Santiago de Chile, Chile 3 European Southern Observatory, Karl-Schwarzschild-Str. 2, 85748 Garching b. München, Germany Received 9 April 2014 / Accepted 30 May 2014 ABSTRACT Aims. We investigate the colour–magnitude relation of metal-poor globular clusters, the so-called blue tilt, in the Hydra and Centaurus galaxy clusters and constrain the primordial conditions for star cluster self-enrichment. Methods. We analyse U, I photometry for about 2500 globular clusters in the central regions of Hydra and Centaurus, based on VLT/FORS1 data. We measure the relation between mean colour and luminosity for the blue and red subpopulation of the globular cluster samples. We convert these relations into mass-metallicity space and compare the obtained GC mass-metallicity relation with predictions from the star cluster self-enrichment model by Bailin & Harris (2009, ApJ, 695, 1082). For this we include effects of dynamical and stellar evolution and a physically well motivated primordial mass-radius scaling. Results. We obtain a mass-metallicity scaling of Z ∝ M0.27 ± 0.05 for Centaurus GCs and Z ∝ M0.40 ± 0.06 for Hydra GCs, consistent with the range of observed relations in other environments. We find that the GC mass-metallicity relation already sets in at present-day 5 masses of a few 10 M and is well established in the luminosity range of massive MW clusters like ω Centauri. The inclusion of a primordial mass-radius scaling of star clusters significantly improves the fit of the self-enrichment model to the data. The self- enrichment model accurately reproduces the observed relations for average primordial half-light radii rh ∼ 1−1.5 pc, star formation efficiencies f ∼ 0.3−0.4, and pre-enrichment levels of [Fe/H] − 1.7 dex. The slightly steeper blue tilt for Hydra can be explained either by a ∼30% smaller average rh at fixed f ∼ 0.3, or analogously by a ∼20% smaller f at fixed rh ∼ 1.5 pc. Within the self- enrichment scenario, the observed blue tilt implies a correlation between GC mass and width of the stellar metallicity distribution. We find that this implied correlation matches the trend of width with GC mass measured in Galactic GCs, including extreme cases like ω Centauri and M 54. Conclusions. First, we found that a primordial star cluster mass-radius relation provides a significant improvement to the self- enrichment model fits. Second we show that broadened metallicity distributions as found in some massive MW globular clusters may have arisen naturally from self-enrichment processes, without the need of a dwarf galaxy progenitor. Key words. supernovae: general – globular clusters: general – galaxies: star clusters: general – stars: formation 1. Introduction been nicknamed the blue tilt, and has also been detected through ground-based imaging (Forte et al. 2007; Wehner et al. 2008; 1.1. Colour magnitude relation of extragalactic globular Park 2012). The first strong hint of the existence of such a clusters colour-magnitude relation of GCs was reported in Dirsch et al. (2003), who found that the colour distribution of the brightest The broadband colour distribution of globular clusters (GCs) ex- GCs in NGC 1399 becomes broad and unimodal. Direct evi- hibits a strong bimodality especially in globular cluster systems dence from spectroscopic observations was recently reported for of massive galaxies (e.g. Gebhardt & Kissler-Patig 1999; Kundu M 31 GCs (Schiavone et al. 2013). & Whitmore 2001,Larsenetal.2001), which is often interpreted Interpreted as a mass-metallicity relation, the blue tilt is as a result of star formation and galaxy assembly processes in the equivalent to a scaling with mass of Z ∝ M0.3−0.7,thatde- early universe (see the review of Brodie & Strader 2006). In the pends on environment. Results have so far indicated that the last decade, Hubble Space Telescope (HST) and ground-based trend sets in already at masses slightly below a million so- 5 wide-field imaging of hundreds of GC systems has provided us lar masses, at several 10 M. The most common interpreta- with very detailed information on bimodality (e.g. Dirsch et al. tion of this colour-metallicity relation is self-enrichment. With 2003; Bassino et al. 2006;Pengetal.2006; Harris et al. 2006, increasing primordial cluster mass (and thus increasing potential 2013; Forbes et al. 2011; Kartha et al. 2014). well depth), a larger fraction of SN II metal ejecta are kept in the One of the surprising outcomes of those studies is a cluster and reprocessed into a second generation of stars (Strader correlation between colour and magnitude for GCs of the & Smith 2008; Bailin & Harris 2009). Furthermore a red tilt, i.e. blue subpopulation (e.g. Harris et al. 2006, 2009a,b; Strader a colour magnitude relation for the red GC subpopulation, has et al. 2006; Mieske et al. 2006, 2010; Spitler et al. 2006; been reported in some cases, but it typically is less significant Humphrey 2009; Cockcroft et al. 2009; Forbes et al. 2010; and weaker in metallicity space (e.g. Harris et al. 2006; Mieske Blom et al. 2012; Usher et al. 2013). This phenomenon has et al. 2006, 2010). Article published by EDP Sciences A105, page 1 of 14 A&A 567, A105 (2014) It is worth noting that colour changes as a function of to a limiting magnitude about 0.5 mag brighter than the GCLF GC mass can also occur as a result of dynamical evolution, via turnover. The usage of the U-band gives us a very broad wave- preferential loss of low-mass stars (Kruijssen & Lamers 2008; length baseline that improves our metallicity resolution com- Anders et al. 2009; Kruijssen 2009). This makes lower-mass pared to other studies. Furthermore, our homogeneous data set GCs bluer than their colour for an unaltered stellar mass func- allows us to perform a robust comparison between the two in- tion, thus qualitatively creating a trend in the direction of the vestigated environments. blue tilt. This effect was investigated for example in Mieske et al. The structure of the paper is as follows. In Sect. 2 we discuss (2010) and particularly in Goudfrooij et al. (2014) who stud- the data reduction and selection of GC candidates. In Sect. 3 ied how it depends on the shape of the Initial Mass Function we measure the mass-metallicity relations of GCs in Hydra and (IMF). However, it is clear that the observed blue tilt can only Centaurus. In Sect. 4 we constrain a star cluster self-enrichment be explained in small part by this effect: the observations indi- model by comparing its prediction to the measured relation. We cate a strengthening of the effect at high cluster masses (Harris focus on the inclusion of previously not considered physical fea- et al. 2006; Mieske et al. 2010), exactly opposite to expectations tures into the model, and on the degeneracy between the in- from dynamical evolution: higher mass clusters are less affected put parameters of the model. We also convert the global mass- by dynamical evolution (Baumgardt & Makino 2003), and thus metallicity relation into a mass-metallicity spread relation, and colour changes from this effect will level off at higher cluster compare this to Milky Way GCs. We provide Summary and masses. Conclusions in Sect. 5. An important aspect to keep in mind is that the cluster-to- cluster scatter of mean metallicity due to local variations in pri- mordial star forming conditions is significant, and will dominate 2. Data smooth internal trends with cluster mass for small samples of 2.1. Observations globular clusters (see e.g. Bailin & Harris 2009). To filter out un- derlying trends like self-enrichment from broadband integrated The data analysed for this paper were all taken in service photometry, one needs large homogeneous data sets of GCs, typ- mode with VLT/FORS1. The I-band photometry was obtained ically more than ∼1000 GCs. For example, for the Milky Way in the course of programmes 065.N-0459 (Hydra, PI Hilker) and no blue tilt is detectable because of the considerable cluster-to- 067.A-0358 (Centaurus, PI Hilker), with 3000 s integration time cluster scatter in mean [Fe/H] and the comparably small sample in I-band per 7 × 7 FORS pointing, at good seeing 0.4−0.7 size (e.g. Strader & Smith 2008). Moreover, the Milky Way GC in seven FORS pointings in Centaurus and Hydra and one back- 6 sample has a rather low upper-mass limit (MωCen = 1.5∗10 M), ground pointing. Those data were already presented in Mieske which is another factor for the non-detectability of the blue tilt et al. (2003, 2005) in an SBF analysis of both clusters. This in our Galaxy. I-band data set also comes along with V-band data taken in the same runs and under similar conditions. The V-band data is not used directly for our blue tilt analysis, but we use the 1.2. Multiple stellar populations in massive Galactic GCs V − I colour distribution to finetune the U − I colour calibra- Almost parallel in time to the discovery of the blue tilt in ex- tion, see Sect. 2.2.1. For the present work, these I-(andV-) data tragalactic GC samples, HST imaging of individual stars in were carefully re-reduced.
Details
-
File Typepdf
-
Upload Time-
-
Content LanguagesEnglish
-
Upload UserAnonymous/Not logged-in
-
File Pages14 Page
-
File Size-