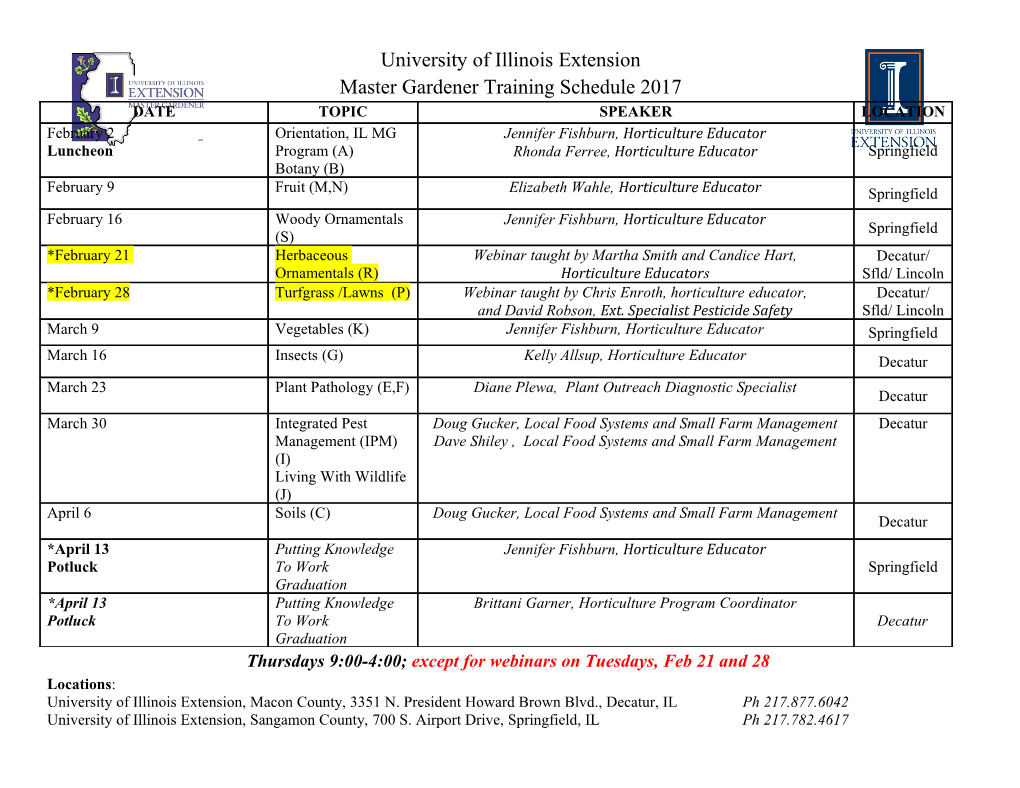
Feasibility-Study for Space-Based Transit Photometry using Mid-sized Nanosatellites by Rachel Bowens-Rubin Submitted to the Department of Earth, Atmospheric, and Planetary Science in partial fulfillment of the requirements for the degree of Masters of Science in Earth, Atmospheric, and Planetary Science at the MASSACHUSETTS INSTITUTE OF TECHNOLOGY June 2012 @ Massachusetts Institute of Technology 2012. All rights reserved. A uthor .. ............... ................ Department of Earth, Atmospheric, and Planetary Science May 18, 2012 C ertified by .................. ........ ........................... Sara Seager Professor, Departments of Physics and EAPS Thesis Supervisor VIZ ~ Accepted by.......,.... Robert D. van der Hilst Schulumberger Professor of Geosciences and Head, Department of Earth, Atmospheric, and Planetary Science 2 Feasibility-Study for Space-Based Transit Photometry using Mid-sized Nanosatellites by Rachel Bowens-Rubin Submitted to the Department of Earth, Atmospheric, and Planetary Science on May 18, 2012, in partial fulfillment of the requirements for the degree of Masters of Science in Earth, Atmospheric, and Planetary Science Abstract The photometric precision needed to measure a transit of small planets cannot be achieved by taking observations from the ground, so observations must be made from space. Mid-sized nanosatellites can provide a low-cost option for building an optical system to take these observations. The potential of using nanosatellites of varying sizes to perform transit measurements was evaluated using a theoretical noise budget, simulated exoplanet-transit data, and case studies to determine the expected results of a radial velocity followup mission and transit survey mission. Optical systems on larger mid-sized nanosatellites (such as ESPA satellites) have greater potential than smaller mid-sized nanosatellites (such as CubeSats) to detect smaller planets, detect planets around dimmer stars, and discover more transits in RV followup missions. Thesis Supervisor: Sara Seager Title: Professor, Departments of Physics and EAPS 3 4 Acknowledgments I would first like to thank my direct advisor, Mary Knapp, for her time, advice, and continual guidance during every part of this project. Next, I would like to thank my advisor, Sara Seager, and my thesis committee members, Richard Binzel and Josh Winn, for their discussion and feedback on my research. Additionally, I would like to thank Jane Conner for teaching me the ins and outs of technical writing and for providing constant encouragement over the past four years. I would also like to acknowledge my labmates Brice Demory, Julien de Wit, Re- becca Jensen-Clem, Luyao Li, Stephen Messenger, Leslie Rogers, and Matt Smith for their consultations, for supplying me with numerous resources, and for helping me prepare for my defense presentation. Lastly, I would like to salute my friends and fellow goggleheads, Zach Barryte who taught me how to make pretty figures using Illustrator and Danbee Tauntaun Kim for her late night reassurance and support. 5 6 Contents 1 Science Background 15 1.1 Purpose of Study: To determine the viability of an ESPA satellite for measuring exoplanet transits ... ........ ........ 15 1.2 Current Methods for Detecting Exoplanets .. ...... ....... 16 1.3 Measuring Exoplanets via the Transit Method ........ 16 1.3.1 Brightness Over Time: Implications of the shape of the light curve. ........... .. .. .. .. .... 16 1.3.2 Probability of Finding an Exoplanet by Transit . 18 1.3.3 The Photometric Precision Threshold for Detecting a Transit- ing Planet ... ....... ....... ....... ..... 21 1.4 Mathematically Modeling the Shape of a Light Curve .... ..... 22 1.4.1 Estimating the Overall Shape by Deriving the Eclipsed Area . 22 1.4.2 Complications to the Overall Shape: Disconformities in the flux emitted from the solar disc ... ....... ....... 25 1.4.3 Modeling the Change in Flux, Including the Effect from Limb D arkening ........ .......... ........... 27 2 Small Satellites: a lower cost option for space missions 29 2.1 Cubesats: a standardized pico- and nanosatellite system .. ..... 30 2.1.1 ExoplanetSat: a 3U CubeSat capable of observing exoplanet transits ... ..... ..... ...... ..... ..... 32 2.2 ESPA Satellites: a larger standardized satellite system alternative .. 33 2.2.1 Castor: an ESPA spacecraft with the potential to be repurposed 35 7 3 Creating a Noise Budget for Space-Based Transit Observations 37 3.1 Important Choices for Engineering Systems. .. .. .. .. 3 7 3.1.1 Detector: CCD versus CMOS . ... .. .. 37 3.1.2 Optical System .. ... ... ... ... .. 39 3.1.3 Orbit Type: Low Earth Orbit . .. .. .. 39 3.1.4 Temperature Fluctuations: Using a Thermal Control System 39 3.2 Contributing Sources of Error ... .. .. .. .. .. .. .. 4 0 3.2.1 Shot Noise .... ..... .. .. .. .. .. .. .. 4 0 3.2.2 Dark Current ... ..... .. .. .. .. .. .. .. .. 4 2 3.2.3 Read Noise .. ..... .. .. .. .. .. .. .. .. 4 3 3.2.4 Jitter Noise .. ... .... .. .. .. .. .. .. .. .. .. 4 4 3.2.5 Quantization Noise..... .. .. .. .. .. .. .. 4 6 3.2.6 Background Light .. ... .. .. .. .. .. .. .. 4 7 3.2.7 Gain Drift ..... ..... .. .. .. .. .. .. .. 4 7 3.2.8 Noise Sources Not Modeled .. .. .. .. .. .. .. 4 8 3.3 Calculating the Total Noise . .. .. .. .. .. .. 4 8 3.3.1 Total Error Budget..... .. .. .. .. .. .. .. 4 8 3.3.2 Effect of Aperture Size, Target Magnitude, and Time Resolu- tion on Total Noise ............. .. .. 50 4 Simulating Data of Exoplanet Transits Collected from Space-Based Missions 53 4.1 The Overall Shape of the Light Curve .. .... ... ... ... ... 53 4.2 Adding Noise Consistent with the Error Budget ... ... ... .. 54 4.3 Gaps Due to Orbit Day and Night .... ... ... ... .... ... 55 4.4 Stacking Transits and Binning to Improve the Photometric Precision 55 5 Radial Velocity Follow-up and Transit Survey Case Studies 59 5.1 Determining the Frequency of Observable Transits .. ... ... .. 60 5.1.1 Selecting Targets and Finding Transit Windows . ... .. .. 60 5.1.2 Orbit Model and Observable Windows . .. ... .. ... .. 61 8 5.1.3 Observable-Transit Windows: When Transits can be Observed 62 5.2 Modeling Data of Transits Using the Results of the Frequency Study 65 5.3 Probability of the Mission Measuring a Transit: ESPA Conditions Compared with ExoSat Conditions ....... ........... 67 5.3.1 Selecting Planets for a RV survey . ...... ..... 67 5.3.2 Probability of Discovering Transits of Known-RV Planets .. 69 5.4 Beyond RV follow-up: Expected Results of a Transit Survey using a Mid-sized Nanosatellite around G and K stars .... ........ 71 5.4.1 Number of Non-variable G and K Stars which could be Monitored 71 5.4.2 Expected Number of Planets to Discover in a Transit Survey Using a Mid-sized Nanosatellite . 73 5.4.3 Transit-Survey Mission Lifetime Requirement .... ..... 74 6 Discussions and Recommendations Related to the Future Use of Mid-sized Nanosatellites to Measurements of Exoplanets 79 6.1 Size Limiting Factors for Building Satellites to Perform Photometry 79 6.2 Constellation of CubeSats verses One ESPA-Class Satellite ... 81 6.2.1 Advantages of a Constellation of CubeSats . ....... 81 6.2.2 Advantages of Using One ESPA-Class Satellite . 82 A Summary of Planet Candidates Used in Determining the Probability of Discovering a Transit in an RV Followup Mission 89 9 10 List of Figures 1-1 Exoplanet Transit Alignment . ............. 18 1-2 Planet Transiting a Star Correlated with the Measured Brightness . 19 1-3 Transit Duration, Transit Depth, and Duration of Ingress/Egress .. 20 1-4 Side View and Observer's View of the Planet's Orbit ......... 21 1-5 Top View and Observer's View of the Planet's Orbit . 23 1-6 Overlapping Stellar and Planetary Disc .... ............ 24 1-7 Limb Darkening Coefficients ... .................... 26 1-8 Area of a Partial Annulus, used for calculating the change of flux with lim b darkening ...... ............ ........... 28 2-1 1U, 2U, and 3U CubeSat Designs ..... 30 2-2 Poly-Picosatellite Orbital Deployer Schematic and Inside ....... 31 2-3 Diagrams of the ExoSat Design ...... 33 2-4 EELV-ESPA system ...... ............. ........ 34 2-5 Model of an ESPA ring with Six Docking Rings ............ 34 2-6 Model of Castor, an ESPA satellite designed at MIT . ........ 35 3-1 Total Noise Budget ... ......................... 41 3-2 Spot Motion on the Detector ............. .......... 44 3-3 Pixel Correlation Time .......... ............. ... 45 3-4 Quantization Noise: Input and Output signals into the ADC ..... 46 3-5 Sources of Error for Time Resolution of One Hour and Aperture Size of 30-cm ..................... ............. 49 11 3-6 Total Noise Estimate varying by Aperture Size for a collection duration of 20 m inutes ..... .... ..... ..... .... ..... ... 51 3-7 Aperture Needed to detect an Earth-Sized Planet ... ..... ... 51 4-1 Shape of 55 Cancri E Transit ...... ............. ... 54 4-2 55 Cancri E with Error . ...... ..... ..... .... ..... 55 4-3 55 Cancri E Transit with Orbit Day and Night Data ... ...... 56 4-4 55 Cancri E Transit with only Orbit Night Data .... ..... 56 4-5 Stacking Transits ... ............. ............ 57 4-6 Ten 55 Cancri E transits Stacked and Binned .... .... ..... 58 5-1 Predicted Transit, Observational, and Observational-Transit Windows for H D 20794 . ........ ........ ........ ....... 62 5-2 Observable Window Zoomed . ...... ..... .... ..... .. 63 5-3 All Observable-Transit Windows . ...... ...... ...... .. 65 5-4 Data Collected from HD20794c Observational-Transit Windows .. 66 5-5 Data Collected from
Details
-
File Typepdf
-
Upload Time-
-
Content LanguagesEnglish
-
Upload UserAnonymous/Not logged-in
-
File Pages97 Page
-
File Size-