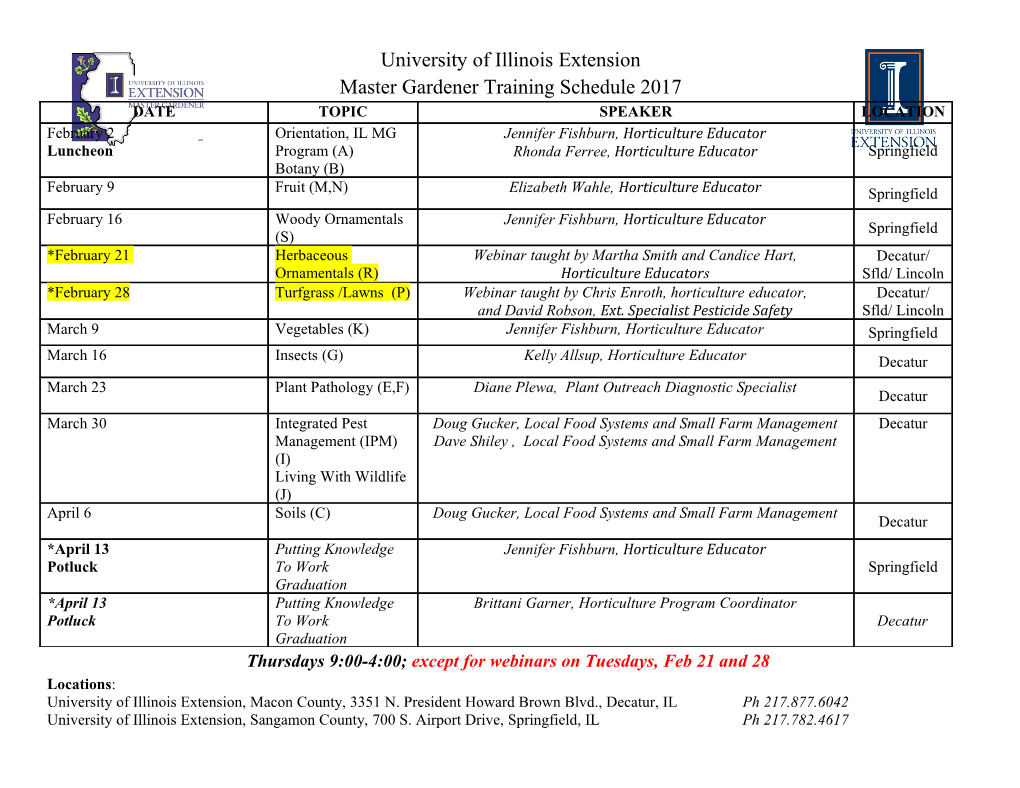
This Accepted Manuscript has not been copyedited and formatted. The final version may differ from this version. A link to any extended data will be provided when the final version is posted online. Research Articles: Systems/Circuits Schaffer collateral inputs to CA1 excitatory and inhibitory neurons follow different connectivity rules Osung Kwon1, Linqing Feng1, Shaul Druckmann2 and Jinhyun Kim1,3 1Center for Functional Connectomics, Brain Science Institute, Korea Institute of Science and Technology (KIST), Korea 2Janelia Farm Research Campus, Howard Hughes Medical Institute, USA 3Division of Bio-Medical Science & Technology, KIST-School, University of Science and Technology, Korea DOI: 10.1523/JNEUROSCI.0155-18.2018 Received: 20 January 2018 Revised: 12 March 2018 Accepted: 19 April 2018 Published: 4 May 2018 Author Contributions: J.K. conceived the project and designed the experiments. O.K. and J.K. performed the molecular biology, animal surgery, immunostaining and imaging. L.F. and S.D. designed and performed all statistical data analysis. L.F., S.D., and J.K. wrote the paper. Conflict of Interest: The authors declare no competing financial interests. We thank Dr. Yong Chul Bae and Jin Young Bae for their assistance with immuno-EM. This work was supported by the KIST Institutional Program (Project No. 2E27850). The authors declare no completing financial interests. Correspondence should be addressed to Jinhyun Kim, Center for Functional Connectomics (CFC), L7-7205, Brain Science Institute at the Korea Institute of Science & Technology (KIST), 39-1 Hawolgokdong, Seongbukgu, Seoul 02792, Korea; phone: +82-2-958-7225, [email protected] Cite as: J. Neurosci ; 10.1523/JNEUROSCI.0155-18.2018 Alerts: Sign up at www.jneurosci.org/cgi/alerts to receive customized email alerts when the fully formatted version of this article is published. Accepted manuscripts are peer-reviewed but have not been through the copyediting, formatting, or proofreading process. Copyright © 2018 the authors 1 Schaffer collateral inputs to CA1 excitatory and inhibitory neurons follow different 2 connectivity rules 3 4 [Short title] Different synapse connectivity rules in excitatory and inhibitory neurons 5 6 Osung Kwon1,4, Linqing Feng1,4, Shaul Druckmann2, and Jinhyun Kim1,3* 7 8 1Center for Functional Connectomics, Brain Science Institute, Korea Institute of Science and 9 Technology (KIST), Korea; 2Janelia Farm Research Campus, Howard Hughes Medical Institute, 10 USA; and 3Division of Bio-Medical Science & Technology, KIST-School, University of Science 11 and Technology, Korea 12 4Co-first author 13 *Correspondence should be addressed to Jinhyun Kim, Center for Functional Connectomics 14 (CFC), L7-7205, Brain Science Institute at the Korea Institute of Science & Technology (KIST), 15 39-1 Hawolgokdong, Seongbukgu, Seoul 02792, Korea; phone: +82-2-958-7225, 16 [email protected] 17 18 19 x 30 pages 20 x 7 Figures, 1 Extended Table, 1 Extended Movie 21 x 186 words in Abstract, 641 words in Introduction, and 1597 words in Discussion 22 23 24 Acknowledgements 25 We thank Dr. Yong Chul Bae and Jin Young Bae for their assistance with immuno-EM. This 26 work was supported by the KIST Institutional Program (Project No. 2E27850). The authors 27 declare no completing financial interests. 28 29 30 31 32 1 33 Abstract 34 35 36 Neural circuits, governed by a complex interplay between excitatory and inhibitory neurons, are 37 the substrate for information processing, and the organization of synaptic connectivity in neural 38 network is an important determinant of circuit function. Here, we analyzed the fine structure of 39 connectivity in hippocampal CA1 excitatory and inhibitory neurons innervated by Schaffer 40 collaterals (SCs) using mGRASP in male mice. Our previous study revealed spatially structured 41 synaptic connectivity between CA3-CA1 pyramidal cells (PCs). Surprisingly, parvalbumin- 42 positive interneurons (PVs) showed a significantly more random pattern spatial structure. 43 Notably, application of Peters’ Rule for synapse prediction by random overlap between axons 44 and dendrites enhanced structured connectivity in PCs, but, by contrast, made the connectivity 45 pattern in PVs more random. In addition, PCs in a deep sublayer of striatum pyramidale 46 appeared more highly structured than PCs in superficial layers, and little or no sublayer 47 specificity was found in PVs. Our results show that CA1 excitatory PCs and inhibitory PVs 48 innervated by the same SC inputs follow different connectivity rules. The different organizations 49 of fine scale structured connectivity in hippocampal excitatory and inhibitory neurons provide 50 important insights into the development and functions of neural networks. 51 2 52 Significance Statement 53 54 Understanding how neural circuits generate behavior is one of the central goals of neuroscience. 55 An important component of this endeavor is the mapping of fine-scale connection patterns that 56 underlie, and help us infer, signal processing in the brain. Here, using our recently developed 57 synapse detection technology (mGRASP and neuTube), we provide detailed profiles of synaptic 58 connectivity in excitatory (CA1 pyramidal) and inhibitory (CA1 parvalbumin-positive) neurons 59 innervated by the same presynaptic inputs (CA3 Schaffer collaterals). Our results reveal that 60 these two types of CA1 neurons follow different connectivity patterns. Our new evidence for 61 differently structured connectivity at a fine scale in hippocampal excitatory and inhibitory 62 neurons provides a better understanding of hippocampal networks and will guide theoretical and 63 experimental studies. 64 65 3 66 Introduction 67 68 Neuronal circuits exhibit multiple types of precise patterns of connectivity between 69 specialized groups of neurons (DeBello, 2014; Druckmann et al., 2014; Harris and Shepherd, 70 2015; Hofer et al., 2011; Jiang et al., 2015; Kasthuri et al., 2015; Rieubland et al., 2014; Straub et 71 al., 2016). Synaptic connectivity is a central element in shaping neural dynamics and the 72 computational functions it supports. Therefore, it is imperative to map the organization of 73 synaptic connections and the principles underlying this organization. Indeed, much progress has 74 been made toward unveiling the organization of complex neural networks in increasing detail: 75 different cell types, afferent and efferent projections, synaptic connections and more have 76 recently been assessed with new technologies (Bloss et al., 2016; Cadwell et al., 2016; 77 Druckmann et al., 2014; Kasthuri et al., 2015; Oh et al., 2014; Wallace et al., 2017; Zingg et al., 78 2014). It is important to obtain quantitative descriptions of connectivity motifs and network 79 architectures, particularly those of excitatory and inhibitory neurons innervated by the same type 80 of cell. However, such descriptions remain elusive. 81 Recent studies of synaptic connectivity have focused on the spatial distribution of 82 afferent inputs (structured vs random) (Bloss et al., 2016; Druckmann et al., 2014; Kasthuri et 83 al., 2015; J. S. Kim et al., 2014) and have attempted to elucidate basic principles of connectivity, 84 for instance, with reference to Peters’ rule. Peters’ rule, a method proposed to predict synaptic 85 connectivity on the basis of geometric overlaps between axon and dendrite, has been interpreted 86 to provide support for random synaptic connectivity (Braitenberg and Schüz, 1998; Peters and 87 Feldman, 1976). This idea has been extrapolated to a general principle of brain organization and 88 has been used extensively in models of theoretical networks and theories about random 89 connectivity at the cellular and subcellular levels (Markram, 2006). However, when recent 90 studies have directly tested Peters’ rule with advanced technologies, conclusions have been 91 divided between confirming and refuting the concept; therefore, the debates continue (Rees et al., 92 2017). Previous studies, including our own, of postsynaptic dendrites and dendritic subdomains 93 of different neuron types show structured synaptic connectivity at the cellular and branch levels 94 in a way that is not consistent with Peters’ rule (Druckmann et al., 2014; Kasthuri et al., 2015; 95 Mishchenko et al., 2010; Rieubland et al., 2014). Additionally, recent studies with advanced 96 technologies demonstrate that traditionally defined cell types need to be refined by additional 4 97 criteria, such as topological location along principal axes, long-distance projection targets, and 98 comprehensive transcriptomes, and that these newly defined subpopulations exhibit structural 99 and functional differentiations, suggesting subtype-specific connectivity (Danielson et al., 2016; 100 Geiller et al., 2017; S.-H. Lee et al., 2014; Valero et al., 2015). Despite the need for a precise 101 description of subpopulation-specific connectivity, such information is not yet available at fine 102 spatial scales. 103 Here, we contribute to this description with a comprehensive, fine-scale mapping of the 104 spatial synaptic profiles of the main excitatory synaptic Schaffer collateral (SC) inputs onto 105 dorsal hippocampal CA1 inhibitory neurons – parvalbumin-positive interneurons (PVs) – as 106 major target interneurons of SC inputs. To achieve this goal, we used mammalian GFP 107 reconstitution across synaptic partners (mGRASP), as previously described (Druckmann et al., 108 2014; Feng et al., 2012; J. Kim et al., 2012). We found a broad range of spatial structure of 109 synaptic connections
Details
-
File Typepdf
-
Upload Time-
-
Content LanguagesEnglish
-
Upload UserAnonymous/Not logged-in
-
File Pages39 Page
-
File Size-