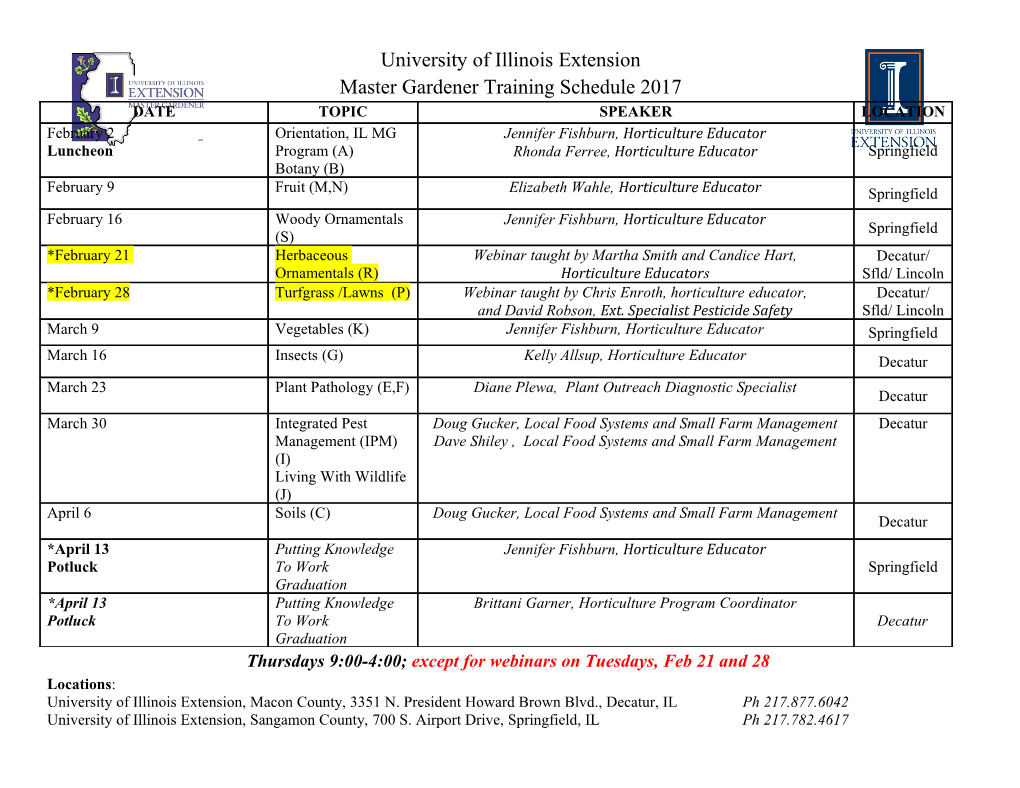
Ancient habitable environments preserved in the rock record Determining whether environmental and chemical conditions have ever been suitable to sustain life on Mars as we know it on Earth has been a focus of recent Mars missions. Significantly, the potential for habitable environments during a time window in Mars' ancient past (the Noachian- Hesperian transition) has now been identified at Yellowknife Bay in Gale crater by the Mars Science Laboratory (MSL) science team. This consensus is based upon comprehensive sedimentological, mineralogical, and geochemical observations, and includes: 1) unambiguous evidence for fluvial transport of coarse-grained sediments, in concert with 2) deltaic deposits confirming the presence of a standing body of water together with laminated mudstones – also indicative of quiescent water deposition; 3) clay mineral detection and lack of chemical precipitates, confirming high water/rock ratio exposure in low-salinity water of near-neutral pH; 4) the presence of key chemical elements used by biology, including native carbon; and 5) the detection of nutrients such as nitrates and energy sources for microbial metabolism such as minerals in various oxidation states. Estimates of sedimentation rates in standing water are used to infer that a hydrologic cycle involving precipitation, runoff, infiltration, groundwater migration, and evaporation would have persisted for perhaps millions of years. Together, these observations make a compelling case for site-specific habitable environments in Mars' ancient past, which will help inform life-search strategies moving forward. It has become apparent in recent years, from the results of extended missions on multiple ground-based and orbital platforms, that early aqueous environments varied considerably in character both spatially and temporally, potentially extending well beyond the Noachian-Hesperian transition. Conditions may have alternated between wet/neutral and dry/acidic, with water persisting in some regions significantly longer than others. Spectral signatures for clay minerals are detected in Noachian crustal rocks throughout both hemispheres, demonstrating abundant high water/rock ratios for those early environments. Environments conducive to phyllosilicate-producing, mineral-water interactions include both hydrothermal and low-temperature water systems. In addition to clay minerals, localized lacustrine sedimentary deposits have preserved carbonates, sulfates, and chlorides. An in-situ effort to study ancient aqueous alteration processes in the Noachian environment of the Endeavour crater rim, is being conducted by the Mars Exploration Rover (MER) Opportunity rover in concert with Compact Reconnaissance Imaging Spectrometer for Mars (CRISM) spectroscopic analysis of altered rocks from orbit. This approach is helping to characterize the geologic and geochemical context for Noachian aqueous alteration processes across the globe. Considerable evidence of an ice-melt-based hydrology in the post-Noachian is found from orbit in the form of widespread, paleolake-associated, fresh shallow valleys throughout the mid latitudes of both hemispheres. Evidence argues for their formation during a period of global climate adjustment. History of the infilling of Gale crater and its exhumation to form Mount Sharp. A north-south transect of the current topography, featuring the high southern rim, the crater’s central peak at nearly equal elevation, the slightly lower false summit of Mount Sharp, and the much lower northern rim. The northern rim of Gale crater is assumed to have this lower elevation because it straddles the dichotomy boundary. The dotted box represents the part of the crater that is illustrated in (C) and (D). (B) Early infilling of the crater during the aqueous period. The dashed line represents the modern topography, and the solid line represents the original post-impact topography, derived from the profiles of other craters of similar size and with central peaks of similar height The infilling of the crater was driven by erosion of the northern rim and the central peak, which generated sediment that was transported downslope, fining along the way due to hydraulic segregation of grain size. Crater diameter-depth relationships suggest that an additional 1 to 2 km of crater-infilling strata (pattern with question marks) may lie beneath the present-day surface (figs. S9 and S10). (C) During the subsequent dry period, the bulk of Mount Sharp formed via accumulation of eolian facies, very generally defined. The mass of Mount Sharp exerted a gravitational force that caused compaction of previously deposited strata, resulting in rotation of those strata downward relative to the rigid central peak (figs. S7 and S8). (D) Wind-driven erosion resulted in the exhumation of the crater-filling strata. Curiosity landed on nearly the lowest point of the exhumation surface, where fluvial-deltaic deposits were exposed, and then drove uphill to the south and across a facies transition into lacustrine sediments of the Murray formation. Figure and caption from Grotzinger et al. (2015); see below. Relevant references: Crumpler, L. S., R. E. Arvidson, D. W. Mittlefehldt, B. L. Jolliff, W. H. Farrand, V. Fox, M. P. Golombek, and A. S. Team (2016), Opportunity, geologic and structural context of aqueous alteration in noachian outcrops, Marathon Valley and rim of Endeavour crater, LPSC XLVII, The Woodlands, TX, Abstract #2272. Ehlmann, B. L., and C. S. Edwards (2014), Mineralogy of the martian surface, Annual Review of Earth and Planetary Sciences, 42, 291-315, doi:10.1146/annurev-earth-060313-055024. Wilson, S. A., A. D. Howard, J. E. Moore, and J. A. Grant (2016), A cold-wet middle-latitude environment on Mars during the Hesparian-Amazonian transition: Evidence from northern Arabia valleys and paleolakes, Journal of Geophysical Research: Planets, 121(9), 1667-1694, doi:10.1002/2016JE005052. Grotzinger, J. P., et al. (2015), Deposition, exhumation, and paleoclimate of an ancient lake deposit, Gale crater, Mars, Science, 350(6257), doi:10.1126/science.aac7575. Szabo, T., G. Domokos, J. P. Grotzinger, and D. J. Jerolmack (2015), Reconstructing the transport history of pebbles on Mars, Nature Communications, 6, doi:10.1038/ncomms9366. Vasavada, A. R., et al. (2014), Overview of the Mars Science Laboratory mission: Bradbury Landing to Yellowknife Bay and beyond, Journal of Geophysical Research-Planets, 119(6), 1134-1161, doi:10.1002/2014je004622. Williams, R.M., Grotzinger, J.P., Dietrich, W.E., Gupta, S., Sumner, D.Y., Wiens, R.C., Mangold, N., Malin, M.C., Edgett, K.S., Maurice, S. and Forni, O. (2013), Martian fluvial conglomerates at Gale crater, Science, 340(6136), pp.1068-1072, DOI: 10.1126/science.1237317. Vaniman, D.T., Bish, D.L., Ming, D.W., Bristow, T.F., Morris, R.V., Blake, D.F., Chipera, S.J., Morrison, S.M., Treiman, A.H., Rampe, E.B. and Rice, M. (2014), Mineralogy of a mudstone at Yellowknife Bay, Gale crater, Mars. Science, 343(6169), DOI: 10.1126/science.1243480. .
Details
-
File Typepdf
-
Upload Time-
-
Content LanguagesEnglish
-
Upload UserAnonymous/Not logged-in
-
File Pages3 Page
-
File Size-