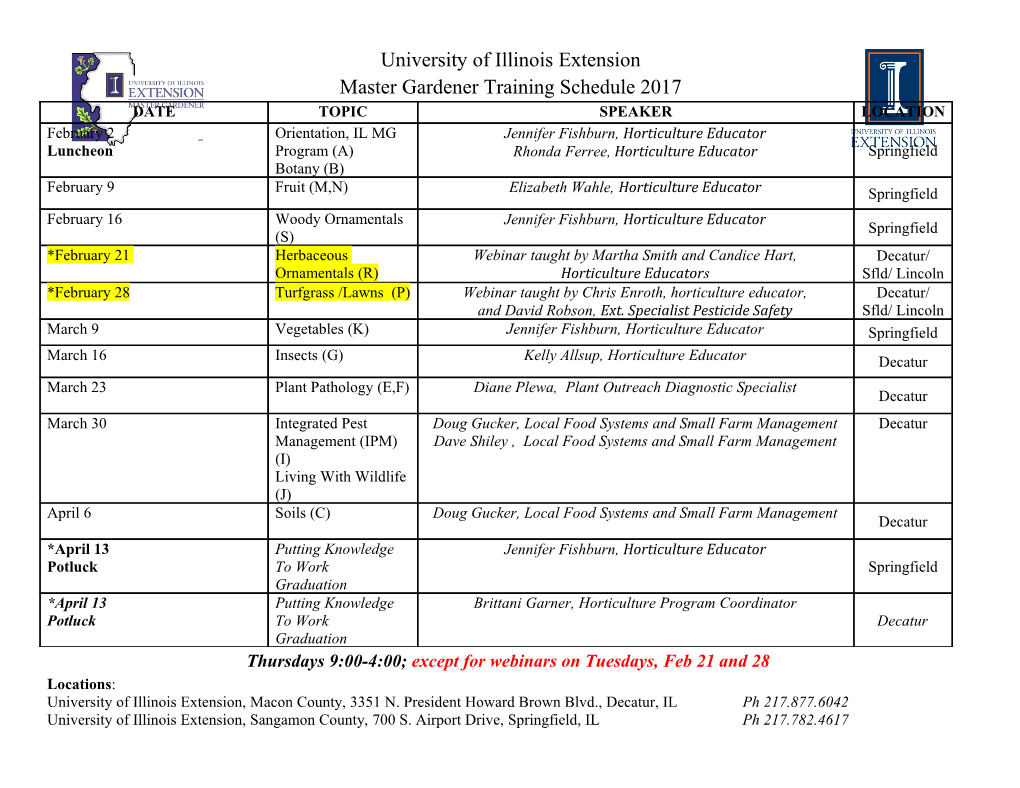
UC Berkeley UC Berkeley Electronic Theses and Dissertations Title Plasma Diagnostics and Plasma-Surface Interactions in Inductively Coupled Plasmas Permalink https://escholarship.org/uc/item/0hn5z4f1 Author Titus, Monica Joy Publication Date 2010 Peer reviewed|Thesis/dissertation eScholarship.org Powered by the California Digital Library University of California Plasma Diagnostics and Plasma-Surface Interactions in Inductively Coupled Plasmas by Monica Joy Titus A dissertation submitted in partial satisfaction of the requirements for the degree of Doctor of Philosophy in Chemical Engineering in the Graduate Division of the University of California, Berkeley Committee in charge: Professor David B. Graves, Chair Professor Roya Maboudian Professor Michael A. Lieberman Spring 2010 Plasma Diagnostics and Plasma-Surface Interactions in Inductively Coupled Plasmas Copyright 2010 by Monica Joy Titus Abstract Plasma Diagnostics and Plasma-Surface Interactions in Inductively Coupled Plasmas By Monica Joy Titus Doctor of Philosophy in Chemical Engineering University of California, Berkeley Professor David B. Graves, Chair The semiconductor industry’s continued trend of manufacturing device features on the nanometer scale requires increased plasma processing control and improved understanding of plasma characteristics and plasma-surface interactions. This dissertation presents a series of experimental results for focus studies conducted in an inductively coupled plasma (ICP) system. First novel “on-wafer” diagnostic tools are characterized and related to plasma characteristics. Second, plasma-polymer interactions are characterized as a function of plasma species and processing parameters. Complimentary simulations accompany each focus study to supplement experimental findings. Wafer heating mechanisms in inductively coupled molecular gas plasmas are explored with PlasmaTempTM, a novel “on-wafer” diagnostic tool. Experimental wafer measurements are TM obtained with the PlasmaTemp wafer processed in argon (Ar) and argon-oxygen (Ar/O2) mixed plasmas. Wafer heating mechanisms were determined by combining the experimental measurements with a 3-dimensional heat transfer model of the wafer. Comparisons between pure Ar and Ar/O2 plasmas demonstrate that two additional wafer heating mechanisms can be important in molecular gas plasmas compared to atomic gas discharges. Thermal heat conduction from the neutral gas and O-atom recombination on wafer surface can contribute as much as 60 % to wafer heating under conditions of low-energy ion bombardment in molecular plasmas. Measurements of a second novel “on-wafer” diagnostic sensor, the PlasmaVoltTM, were tested and validated in the ICP system for Ar plasmas varying in power and pressure. Sensor measurements were interpreted with a numerical sheath simulation and comparison to scaling laws derived from the inhomogeneous sheath model. The study demonstrates sensor measurements are proportional to the RF-current through the sheath and the scaling is a function of sheath impedance. PlasmaVoltTM sensor measurements are proportional to the square root of the plasma density at the plasma-sheath interface, one-fourth root of the electron temperature, and one-fourth root of the RF bias voltage under conditions where the sheath is predominantly capacitive. When the sheath impedance becomes increasingly resistive, the sensor measurements deviate from the scaling law and tend to be directly proportional to the plasma density. 1 Vacuum ultraviolet (VUV) emissions in Ar ICPs are characterized and the chemical and physical modifications to 193 nm photoresist (PR) polymer materials processed in Ar ICPs are investigated. Fourier transform infrared (FTIR) transmission measurements as a function of VUV photon fluence demonstrate that VUV-induced bond breaking occurs over a period of time. A numerical model demonstrates that VUV photons deplete near-surface O-containing bonds, leading to deeper, subsequent penetration and more bond losses, while the remaining near- surface C–C bonds absorb the incident radiation and slow VUV photon penetration. The roughening mechanism of blanket and patterned 193 nm PR samples are explored in a well characterized Ar ICP. FTIR and atomic force microscopy (AFM) analysis of plasma processed 193 nm PR suggests that ion-induced generation of a graphitized layer at high energies, combined with VUV bulk modification of 193 nm PR may initiate PR roughening. The roughness of blanket samples increases as a function of VUV fluence, ion energy, and substrate temperature. Line width roughness (LWR) measurements of patterned samples demonstrate a similar trend suggesting that LWR may correlate with surface roughness of patterns. The results are compared to PR studies previously conducted in an ultra-high vacuum beam system demonstrating that the vacuum beam system is a useful tool that can deconvolute and simplify complex plasma systems. 2 To my mom, dad, and brother. i Table of Contents List of Figures ……………………………………………………………………………... vi List of Tables ……………………………………………………………………………... xi Acknowledgements ………………………………………………………………………... xiii 1 Introduction ……………………………………………………………………………. 1 1.1 The Semiconductor Industry ……………………………………………………… 1 1.2 Photolithography ………………………………………………………………….. 2 1.3 Plasma Discharges ………………………………………………………………... 4 1.4 Plasma & Wafer Diagnostics – Metrology ……………………………………….. 5 1.4.1 Wafer Diagnostics ………………………………………………………… 5 1.4.2 Plasma Diagnostics ……………………………………………………….. 6 1.5 Numerical Modeling ……………………………………………………………… 8 1.6 Thesis Outline …………………………………………………………………….. 8 1.6.1 “On-wafer” Sensors ………………………………………………………. 8 1.6.2 193 nm Photoresist ………………………………………………………... 9 2 The Inductively Coupled Plasma System & Diagnostic Tools ……………………… 10 2.1 Abstract …………………………………………………………………………… 10 2.2 Inductively Coupled Plasma Chamber ……………………………………………. 10 2.3 Sample Holder ……………………………………………………………………. 11 2.4 Quartz Crystal Microbalance ……………………………………………………... 11 2.5 Langmuir Probe …………………………………………………………………… 14 2.6 Ion Current Probe …………………………………………………………………. 15 2.7 Ion Mass Spectrometer ……………………………………………………………. 16 2.8 Appearance Potential Mass Spectrometer ………………………………………… 17 2.9 Optical Emission Spectrometer …………………………………………………… 18 2.10 Vacuum Ultraviolet Spectrometer ………………………………………………... 18 3 Argon Plasma Characterization ……………………………………………………… 21 3.1 Abstract …………………………………………………………………………… 21 3.2 Langmuir Probe …………………………………………………………………… 21 ii 3.2.1 Power and Pressure Effects ……………………………………………….. 22 3.2.2 Plasma Perturbation Effects ………………………………………………. 23 3.2.3 Scaling Experimental Measurements to Substrate Surfaces ……………… 24 3.3 Ion Current Probe …………………………………………………………………. 24 3.3.1 Effects of Power and Pressure ……………………………………………. 24 3.3.2 Effects of Bias Voltage …………………………………………………… 25 3.3.3 Effects of Surface Area …………………………………………………… 26 3.4 Ion Mass Spectrometry …………………………………………………………… 27 3.5 Optical Emission Spectrometry …………………………………………………... 29 3.5.1 Plasma Stability Measurements …………………………………………... 29 3.5.2 Monitoring Plasma Resonance and Metastable States ……………………. 30 3.6 Vacuum Ultraviolet Spectrometry ………………………………………………... 32 4 Wafer Heating Mechanisms in a Molecular Gas, Inductively Coupled Plasma …... 35 4.1 Abstract …………………………………………………………………………… 35 4.2 Introduction ……………………………………………………………………….. 35 4.3 Experimental Methods ……………………………………………………………. 36 4.3.1 The “on-wafer” sensor: PlasmaTempTM ………………………………….. 36 4.3.2 Experimental Apparatus & Diagnostic Tools …………………………….. 37 4.3.3 Experimental Conditions ………………………………………………….. 37 4.4 PlasmaTempTM Thermal Model …………………………………………………... 39 4.5 Results & Discussion ……………………………………………………………... 42 4.5.1 Wafer Heating Mechanisms in Ar Plasmas ………………………………. 42 4.5.2 Wafer Heating Mechanisms in Ar/O2 Plasmas …………………………… 43 4.5.3 Wafer Cooling Mechanisms ………………………………………………. 47 4.6 Conclusion ………………………………………………………………………... 49 5 “On-Wafer” Voltage Sensor Analysis in an Inductively Coupled Plasma ………… 50 5.1 Abstract …………………………………………………………………………… 50 5.2 Introduction ……………………………………………………………………….. 50 5.3 Experimental Methods ……………………………………………………………. 52 5.3.1 Experimental Apparatus & Diagnostic Tools …………………………….. 52 5.3.2 The “on-wafer” sensor: PlasmaVoltTM ……………………………………. 53 5.3.3 Experimental Conditions ………………………………………………….. 54 5.4 Sheath Model Formulation ………………………………………………………... 54 5.5 Results & Discussion ……………………………………………………………... 57 5.5.1 Sheath Model Validation …………………………………………………. 57 5.5.2 Sheath Model Results ……………………………………………………... 59 5.5.3 Validating PlasmaVoltTM Measurements …………………………………. 63 5.5.4 Correlating PlasmaVoltTM Measurements to Plasma Density ……………. 64 5.5.5 Industry Relevant Applications …………………………………………… 68 5.6 Summary & Concluding Remarks ………………………………………………... 69 6 Absolute Vacuum Ultraviolet (VUV) Photon Flux in Inductively Coupled Plasmas and Chemical Modifications of 193 nm Photoresist ……………………… 70 6.1 Abstract …………………………………………………………………………… 70 iii 6.2 Introduction ……………………………………………………………………….. 70 6.3 Experimental Setup ……………………………………………………………….. 71 6.3.1 Inductively Coupled Plasma System ……………………………………… 71 6.3.2 Ultra-high Vacuum Beam System ………………………………………... 72 6.3.3 193 nm Photoresist Characterization ……………………………………... 72 6.4 Results & Discussion ……………………………………………………………... 73 6.4.1 VUV and Ion Flux Characterization ……………………………………… 73 6.4.2 VUV Modification of 193 nm Photoresist ………………………………... 74 6.4.3 Chemical Dosimeter Application …………………………………………. 76 6.5 Conclusion ………………………………………………………………………..
Details
-
File Typepdf
-
Upload Time-
-
Content LanguagesEnglish
-
Upload UserAnonymous/Not logged-in
-
File Pages216 Page
-
File Size-