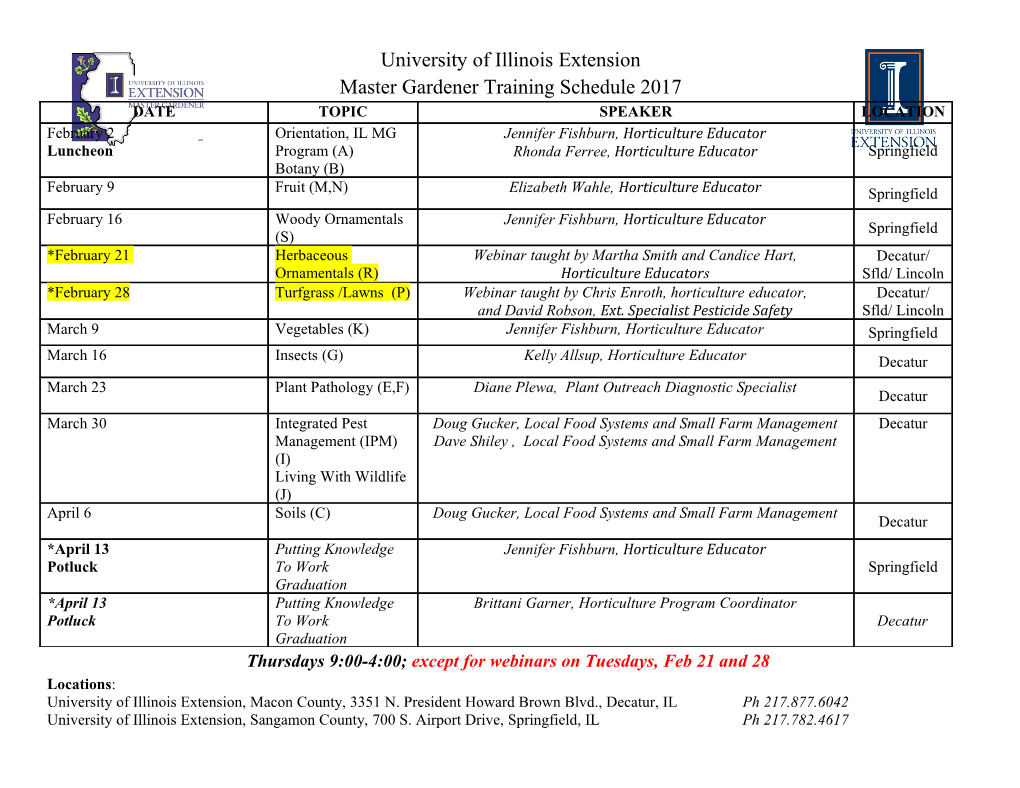
Thermodynamics Rob Iliffe Cartesian Conservation Principle • In Principia Philosophiae (1644) Descartes outlined 3 ‘Laws of Nature’, the first two of which hold • (a) that each thing, as far as is in its power, always remains in the same state (either of rest or of motion), and • (b) that all motion is naturally rectilinear (in a straight line). • Point (a) holds that that no body once in motion could come to rest of its own accord, nor could a resting body start to move of its own accord. • (c) A third law posits that after a collision between two bodies, the combined sum (m1v1 +m2v2) of the ‘size’ and ‘speed’ of each body is conserved, and this applied throughout the cosmos. Theological foundations of conservation theory • Descartes claimed in the second part of the Principia that God preserved a constant amount of matter and motion in recreating the world from one moment to the next. • In the ‘Queries’ to Opticks, Newton argued that the world was tending to run down as a result of elastic collisions, • and so God periodically needed to intervene in his Creation. • In his correspondence with Newton’s ally Samuel Clarke in 1715-16, Leibniz responded that this was an impoverished view of God, • Which implied that he was a poor craftsman. Conservation Matters • Eighteenth century scientists were deeply interested in ‘conservation principles’ operating in a broad range of phenomena, • e.g. in the action of machines, combustion, light and particularly heat. • E.g., Lavoisier’s determination in 1780s, using fine precision measurement, that mass was conserved during chemical reactions in a closed system was central to his refutation of phlogiston, while • it was supposed that the heat substance, ‘Caloric’, was also conserved. • German philosophers working in the tradition of Naturphilosophie, claimed that there was an underlying force that was continuously transformed from one aspect to the next while being conserved. Table of new substances with former names (where appropriate), from Lavoisier, Traité Elémentaire de Chimie (1789), translated in to English as Elements of Chemistry. Caloric is second from top. Heat as Motion: Count Rumford • Benjamin Thompson, Count Rumford, was an American-born British soldier and inventor who was a military advisor to the government of Bavaria from 1784-95 (where he was made ‘Reichsgraf’ or Count). • Having spent many years in Munich performing experiments on gunnery and explosives, • and published work on production of heat through motion (friction) in a Philosophical Transactions article of 1798. • Deeply opposed to Caloric Theory (in which heat was an indestructible fluid), he described a number of experiments that he claimed destroyed the doctrine. The Cannon Trial • Rumford immersed a cannon in water and carved out the inside with a boring-tool; the water boiled within 2 and a half hours. • (a) that frictional heat produced by boring the cannon with a blunt instrument was much greater than that with a sharper instrument (and was proportional to the friction generated); • (b) heat could be created ‘indefinitely’ • (c) mechanical work had been converted into heat. • So heat was created, and the theory of caloric was wrong. The Practical World of Heat Engines • The development of Steam engines throughout the Eighteenth Century was central to the development of The Industrial Revolution. • Engines, initially designed by the ironmonger Thomas Newcomen, were used in coal mines to get rid of water as they went deeper, • And later, improved by the Scottish inventor James Watt, they were used to power textile machines in factories • and also drive mass production of items such as coins. Diagram of Newcomen Engine (1712-1880s) – Balance-beam FED transmits action via chain. Boiler releasing steam is at A, beneath cylinder B (open at top), containing Piston P. At each stroke of the piston , the Water Tank C supplies cold water for condensing steam (which forms a vacuum) in cylinder. Condensation process made the engine inefficient, since it cooled the cylinder. Watt, working on a model of the engine at Glasgow, realized that the machine was inefficient because the cylinder had to be re- heated at every stroke. James Watt made a series of improvements in 1763-75, adding a cold separate condensing chamber (‘condenser’) outside the cylinder which condensed steam without cooling the piston and cylinder walls. Watt’s changes doubled fuel efficiency. Nicolas-Léonard Sadi Carnot (1796-1832) • Son of soldier and geometer Lazare Carnot, Napoleon’s Minister of War, who had himself written a work on the conservation of mechanical energy in 1803, proving the impossibility of Perpetual Motion. • Carnot enrolled at Ecole Polytechnique in 1812 and then attended a School of Engineering at Metz. • Studied the theory of gases in Paris, and discussed steam-engines when he visited his father at Magdeburg in 1821, • a Watt engine having been recently used in the area. • In 1822-3 he worked on his great text, Réflexions sur la puissance motrice du feu et sur les machines propres à développer cette puissance, which appeared in 1824. Sadi Carnot (1796-1832) Caloric and the waterfall Analogy • Carnot used theory of caloric, and the Principle of the Conservation of Caloric, to analyse working of an ideal steam-engine. • Assuming any two separate parts of a machine at different temperatures, then the ‘motive force’ of an engine was dependent on • (a) the fall in (i.e. difference between) the temperature of Caloric as it moves from the hotter to the colder body • (b) and the quantity of Caloric within the system – • just as the height and quantity of water determined the power of a waterfall (while the amount of water remained constant). Modern Carnot engine diagram - where an amount of heat QH flows from a high temperature TH furnace through the fluid of the "working body" (working substance) and the remaining heat QC flows into the cold sink TC, thus forcing the working substance to do mechanical work W on the surroundings, via cycles of contractions and expansions. The Carnot Cycle • The work performed by Caloric in one cycle of an engine was independent of the ‘working substance’ • – any fluid or vapour capable of being expanded. • In a real engine, work was wasted or irreversibly ‘lost’ when parts of an engine with different temperatures were in contact. • A perfect engine was therefore one where the difference in temperature between elements of an engine was minimal. • Thus minimizing the work ‘wasted’. • The question was whether some objective or quantitative meaning could be given to the lost work, in terms of the state of the system. Conservation of ‘Force’: Julius Robert Mayer (1814-71) • Mayer was a practising physician who, while surgeon on boat in the tropics, had made a number of important observations about link between the environment and the use of Oxygen by human bodies. • In his 1842 paper ’Remarks on the forces of inorganic nature’ he argued that the work performed in converting organic resources into life, and in expending work through living, • Was evidence of the conservation of some underlying entity, which he called ‘Kraft’ or ‘force’. Mayer and the Conservation of Energy • Mayer came to believe that both vegetables and animals were complex chemical machines, • Which behave no differently from inorganic machines in virtue of transforming resources from one form into another. • Nothing was created by this process, nor was it destroyed. • Mayer was both a theorist and a careful quantitative measurer of phenomena - • In retrospect, he was one of many who had similar ideas about the conservation of some entity X (later termed ‘energy’) that remained stable through numerous transformations. James Prescott Joule (1818-1889) • Son of a brewer near Manchester; • Joule was fascinated by electricity early on, especially by the Voltaic pile and by Faraday’s work on electromotive force. • Joule was concerned about the precise measurement of heat, partly out of an interest in the science of heat, • and partly to improve his factory’s products. • In the late 1830s he worked on means of comparing and quantifying work extracted from various sources, such as burning a unit of coal in a steam-engine or using various metallic combinations in a battery. • His work was much more precise than that of Mayer or anyone else Joule and Caloric Theory • Joule believed that Caloric theory was wrong, and that heat was either produced by work, or lost in various processes. • In 1839 he began a series of experiments on the relationship between heat, electricity and mechanical work. • He submitted a paper to the Phil. Trans. Entitled ‘On the Production of Heat by Voltaic Electricity’, showing that heat produced by the Voltaic Pile was generated by the electric current – • The paper, like others he wrote at the time, was rejected. • The question was whether all of work expended was fully converted into heat, or whether anything was lost in the process. The Mechanical Equivalence of Heat • In 1843 paper he ascertained that there was a unit of work (Joule) that could be defined as the energy required to raise the temperature of a pound of water (0.45 kg) by 1 degree Fahrenheit. • Joule’s famous demonstration of 1845, was essentially a massive refinement of Rumford’s idea, • involved measuring the heat given off by the motion of a revolving paddle in an insulated bucket of water to within 1/200th of a degree. • Conclusion was that motion and heat were mutually interchangeable; • A given amount of work will generate the same amount of heat, as long as work done was completely converted to heat energy. Demonstration of The Mechanical Equivalence of Heat, 1845 James Joule The Idea of Energy • In 1847, William Thomson (later Lord Kelvin) heard Joule discuss his work on the convertibility and mechanical equivalent of heat.
Details
-
File Typepdf
-
Upload Time-
-
Content LanguagesEnglish
-
Upload UserAnonymous/Not logged-in
-
File Pages28 Page
-
File Size-