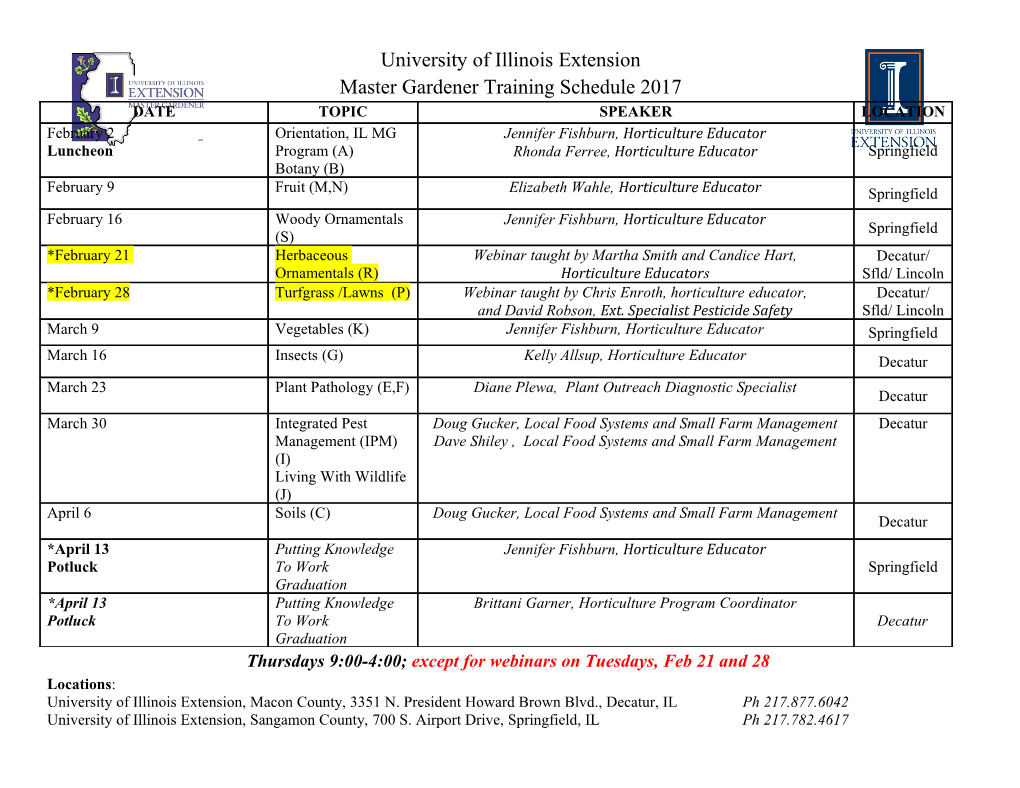
https://doi.org/10.1130/G47282.1 Manuscript received 27 September 2019 Revised manuscript received 1 June 2020 Manuscript accepted 5 June 2020 © 2020 The Authors. Gold Open Access: This paper is published under the terms of the CC-BY license. Published online 21 July 2020 A test of the efficacy of sand saltation for silt production: Implications for the interpretation of loess Steven M. Adams and Gerilyn S. Soreghan School of Geosciences, University of Oklahoma, 100 E. Boyd Street, Norman, Oklahoma 73019, USA ABSTRACT geologically significant parameters. Results ad- Production of the silt that forms loess is attributed to processes operating in both glacial dress the potential for saltation-induced frac- systems (glacial grinding) and sandy deserts (saltation-induced fracturing). However, the ef- turing to produce sufficient silt to form loess, ficacy of saltation for significant silt production is controversial. Understanding the potential and thus bear on the enduring controversy of for silt production in deserts is essential for determining the paleoclimatic significance of loess. “desert versus glacial” origins for geologically To better assess the significance of eolian abrasion for silt production, experimental abrasion significant loess. was conducted in a device designed to simulate sand saltation at sustained storm-wind ve- locities (∼25 m/s). The design differs from previous work in (1) maintaining strong measured METHODS velocities for long duration, (2) removing preexisting silt and utilizing control samples, (3) Sand samples of ∼2 kg each were col- and scaling results to estimate potential for loess accumulation. Scaling experimental rates lected from dune crests at the upwind mar- of production to geologic proportions indicates that eolian abrasion of sand produces insuf- gins of the Algodones (California, USA) and ficient silt to create geologically significant loess deposits. Little Sahara (Oklahoma) dune fields for abrasion experiments (Tables S1 and S2 in INTRODUCTION Previous experiments have studied silt pro- the Supplemental Material1). Samples were Silt composing many of Earth’s major loess duction by processes thought to operate in des- wet sieved to remove grains <250 µm. The deposits is linked to physical grinding from gla- erts, e.g., salt weathering and saltation abrasion <63 µm fraction was measured with a Malvern cial processes (e.g., Pye, 1995; Smalley, 1995; (Kuenen, 1960; Goudie et al., 1979; Pye and Mastersizer 3000 laser particle-size analyzer Assallay et al., 1998; Muhs, 2013). The desert Sperling, 1983; Smith and McGreevy, 1987; (LPSA). The >250 µm fraction was split, with loess hypothesis posits that the silt that forms Whalley et al., 1987; Smith et al., 1991; Wright one half designated as “unwashed,” and the loess originates largely by eolian abrasion of et al., 1998; Wright, 2001; Bullard et al., 2004, other designated as “washed.” Washed sam- sand (e.g., Smalley and Vita-Finzi, 1968; Tsoar 2007; Marshall et al., 2012; Swet et al., 2019). ples were agitated in 5% (NaPO3)6 dispersant and Pye, 1987; Smith et al., 2002; Crouvi et al., Experiments designed to test the efficacy of to remove clay and oxide coatings. For each 2008; Enzel et al., 2010), although Muhs (2013) silt production by intergranular collisions have of the four starting sample types, four 100 g noted that so-called “desert loess” could derive yielded varying results, from negligible (Kue- aliquots were subjected to experimental abra- from deflation of silty sources (e.g., playas) or nen, 1960; Swet et al., 2019) to substantial silt sion, and one 100 g sample was designated silt-rich protoliths. (Whalley et al., 1987; Smith et al., 1991; Wright as a control. The experimental aliquots were Some assert that eolian abrasion plays a large et al., 1998; Wright, 2001; Bullard et al., 2004, abraded for 1, 2, 4, and 8 d. Three additional role in silt production (Smith et al., 2002; Crouvi 2007; Bullard and White, 2005), with the latter Little Sahara washed 4 d samples were abrad- et al., 2008; Enzel et al., 2010). Evaluating the cited as supporting evidence for the hypothesis ed for reproducibility, with processing to re- potential for silt generation by eolian abrasion that sandy deserts produce silt to form loess duce loss (fewer transfers between containers) (defined as intergranular collision during salta- (Smith et al., 2002; Crouvi et al., 2008; Enzel during sample collection and measurement. tion) is fundamental to the use of loess as a pa- et al., 2010). Grain size was measured for unprocessed bulk leoclimate indicator. If desert processes produce Here, we focused on silt (operationally samples using LPSA (Table S2). The starting abundant silt, then loess in the geological record defined as 5–63 µm) experimentally created material was subsampled for grain size and may have originated wholly or in part in deserts. from saltation-induced fracturing of eolian mineralogical analyses (Tables S2 and S3). If deserts do not produce significant silt in the sand under simulated natural wind speeds. The >250 µm fraction used in abrasion was loess size range through eolian abrasion, then These experiments maintained strong storm measured by LPSA. Point counts (n = 400) desert-adjacent loess deposits likely contain silt winds (∼25 m/s) over relatively long intervals on the four types of starting material revealed produced by glaciation or other mechanisms. (up to 8 d), enabling representative scaling of the mineralogy. 1Supplemental Material. Table S1 (GPS coordinates of sample locations), Table S2 (grain-size distribution data; https://doi.pangaea.de/10.1594/pangaea.914301), Table S3 (point-counting data), Table S4 (automated surface observing stations [ASOS] GPS and wind data), Table S5 (scaling methods and detailed calculation), Table S6 (law of wall calculation and plot), and Table S7 (control sample mass loss). Please visit https://doi.org/10.1130/GEOL.S.12555545 to access the supplemental material, and contact [email protected] with any questions. CITATION: Adams, S.M., and Soreghan, G.S., 2020, A test of the efficacy of sand saltation for silt production: Implications for the interpretation of loess: Geology, v. 48, p. 1105–1109, https://doi.org/10.1130/G47282.1 Geological Society of America | GEOLOGY | Volume 48 | Number 11 | www.gsapubs.org 1105 Downloaded from http://pubs.geoscienceworld.org/gsa/geology/article-pdf/48/11/1105/5169306/1105.pdf by guest on 27 September 2021 AB Figure 1. Eolian abrasion device. (A) Schematic diagram of device with Upper Filter 5 µm air-flow directions (blue arrows). Air is pulled through the chamber at ∼25 m/s. Sand blown upward in the chamber 110 5cm descends and collides with upward-blowing sand. (B) Photograph of Vacuum device chamber during Vacuum operation. Arc of blown Air in sand is colliding with Air Out returning sand pulses. HEPA—high-efficiency particulate air. Lower Filter 120 µm HEPA Air Filter Abrasion Device Method for Scaling Results desert was determined using weather station The experimental device (Fig. 1) employed The scaling method converted experimen- data from windy regions (Oklahoma, Texas, two vacuum motors to create an air flow of tal results to a potential maximum of silt pro- and Wyoming [USA], and China; NOAA Na- 3–35 m/s in an inclined (60°), 5-cm-diameter duced by a dune field annually. Maximum tional Climatic Data Center, 2018; Table S4). polycarbonate chamber equipped with steel- annual duration for storm-wind speeds in a The windiest location averaged 322 h above mesh filters at the base (120 µm) and top (5 µm). While grains <5 µm technically could TABLE 1. MASS AND GRAIN-SIZE RESULTS pass through the upper filter, they do not; rath- Silt Grain-size er, they cling to the filter owing to electrostatic Number 5–63 µm mass* vol% mode Clay mass Sand mass Loss mass formation of aggregates >5 µm. Sand placed Sample of days (g) (µm) (g) (g) (g) Algodones washed in the chamber prior to operation settled into a AL-WS-C 0 0.0000 BDL§ 0 99.7778 0.2222 pseudo-dune, wedge shape at the chamber base AL-WS-1D 1 0.0018 41.9 0 99.8301 0.1681 AL-WS-2D 2 0.0019 58.9 0.0034 99.5541 0.4406 (Fig. 1). An antistatic spray prevented particles AL-WS-4D2 4 0.0014 45.6 0.0184 99.6086 0.3716 from clinging to chamber walls. AL-WS-8D 8 0.0037 35.3 0.0296 99.5941 0.3726 Chamber air velocity was measured using Algodones unwashed AL-OR-C 0 0.0000 BDL 0 99.7050 0.2950 a hotwire anemometer with and without sand AL-OR-1D 1 0.0002 BDL 0.0032 99.4031 0.5935 present to determine velocity ranges and asso- AL-OR-2D 2 0.0003 BDL 0.0063 99.7735 0.2199 AL-OR-4D2 4 0.0010 31.1 0.0437 99.5493 0.4060 ciated hop distance of sand under experimental AL-OR-8D 8 0.0011 40.1 0.0476 99.4854 0.4659 conditions. This hop distance was marked on the Little Sahara washed chamber, and the motors were adjusted to main- LS-WS-C 0 0.0000 BDL 0 99.9437 0.0563 LS-WS-1D 1 0.0001 BDL 0.0009 99.8534 0.1456 tain this distance, and the corresponding veloc- LS-WS-2D 2 0.0001 BDL 0.0024 99.8079 0.1896 ity. If the hop distance of the sand diminished, LS-WS-4D1 4 0.0026 51.8 0.0105 99.7641 0.2228 LS-WS-4D2 4 0.0058 43.1 0 99.6951 0.2991 the power was increased. A peak wind speed of LS-WS-4D3 4 0.0039 36.7 0 99.7557 0.2404 25 m/s was selected as representative of severe LS-WS-4D4 4 0.0046 50.1 0 99.7756 0.2198 LS-WS-8D 8 0.0036 45.6 0.0175 99.8391 0.1398 dust storms (Zhou and Wang, 2002), and thus the Little Sahara unwashed most extreme natural conditions.
Details
-
File Typepdf
-
Upload Time-
-
Content LanguagesEnglish
-
Upload UserAnonymous/Not logged-in
-
File Pages5 Page
-
File Size-