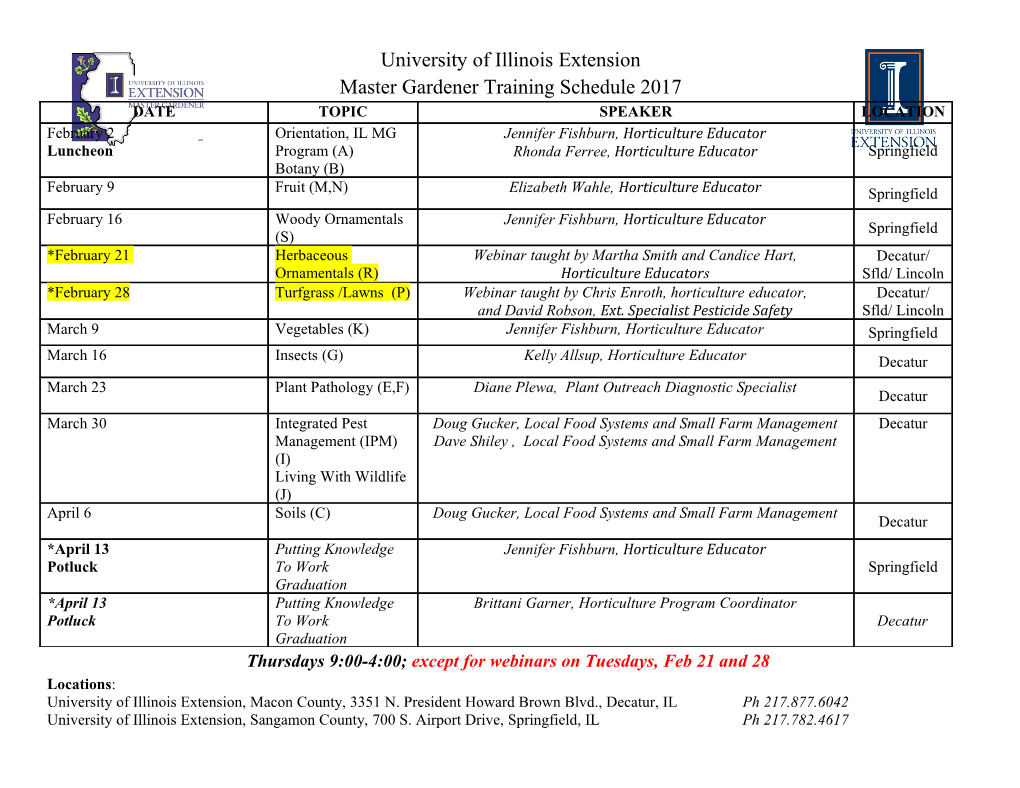
Chapter 6 Target Selection © 2019 Elsevier Inc. All rights reserved. Fig. 6.1 Conceptual, not necessarily successive, stages of targeting. From top to bottom, an axon defasciculates from the main nerve in the region of the target. It enters the target and begins to branch, but is prevented from exiting by a repulsive border. The axon responds to a topographic gradient that promotes branching at the correct location. It then selects a particular layer and finally homes in on particular target cells. © 2019 Elsevier Inc. All rights reserved. 2 Fig. 6.2 Defasciculation is regulated by Beat proteins. (A) Two intersegmental nerve (ISN) motor axons are shown, one that branches off the nerve in the region of its target. (B) In a beat-1a mutant, the motor axon on the right does not defasciculate. (C) The beat-1a mutant phenotype is rescued in a fas II mutant background. © 2019 Elsevier Inc. All rights reserved. 3 Fig. 6.3 Some sympathetic neurons use a change in NT-3 expression to innervate their targets in the ear. (A) Some SCG neurons project to and arborize in the pinna of a normal mouse. (B) In NT-3 knockout mice, these fibers do not invade the pinna. (C) Restoration of targeting by injection of NT-3 into the ear. © 2019 Elsevier Inc. All rights reserved. 4 Fig. 6.4 Innervation of the inner ear is regulated by BDNF (purple) and NT-3 (green). (A) In the wild-type animal, the vestibulo-cochlear ganglion, all of whose neurons express TrkB and TrkC, grow toward the developing inner ear, which has a vestibular and a cochlear primordium. As the system develops and the primordia develop into semicircular canals and a cochlea, the ingrowing axons innervate both parts of the inner ear. (B) In BDNF, NT-3, or TrkB, TrkC double mutants, the inner ear remains uninnervated. (C) In transgenic mice in which BDNF has been knocked into the NT-3 coding region, the cochlear region becomes innervated by the vestibular part of the ganglion. © 2019 Elsevier Inc. All rights reserved. 5 Fig. 6.5 Growth cones change when they enter their target zones. (A) Images from a time-lapse movie of a retinal ganglion cell growing in the optic tract and then crossing (at the dotted line) into the tectum. The simple growth cone becomes much more complex and slows down dramatically as it enters the target. (B) Retinal axons grow on regions high in FGF and slow within the tectum, which has low FGF levels (top); tectum is avoided by retinal axons that misexpress a dominant negative FGF receptor (bottom). (C) Retinal axons slow down and branch when they reach the tectum in control animals (top), but when the pathway is exposed to high levels of FGF-2, the axons keep going and do not innervate the tectum (bottom). © 2019 Elsevier Inc. All rights reserved. 6 Fig. 6.6 Axons branch at pause points. (A) A growth cone pauses, microtubules splayed. (B) The growth cone moves on but leaves behind it a zone where the cytoskeleton remains somewhat disorganized. (C) A branch forms at this zone. © 2019 Elsevier Inc. All rights reserved. 7 Fig. 6.7 Cross wiring of visual signals to the somatosensory and auditory cortex. (A) In a normal control animal, the somatosensory input goes to the ventrobasal nucleus (VBN) of the thalamus and from there to the somatosensory cortex. Retinal input is to the lateral geniculate nucleus (LGN) and the superior colliculus (SC), although neonatally, there is a transient projection to the VBN that is normally eliminated. The visual input to the LGN then goes on to the visual cortex. In an experimental animal in which the superior colliculus, the visual cortex, and the input to the VBM are removed neonatally, the transient retinal projection to the VBN is stabilized and the visual information is thus provided to the somatosensory cortex via the VBN. (B) When similar visual cortical and SC ablations are done and the auditory pathway is cut, the retinal input sprouts into the medial geniculate nucleus (MGN, the normal thalamic target of the auditory input), which projects as usual giving visual physiological properties to the auditory cortex. (C) EphrinA2 and EphrinA5 are expressed at the border between the LGN and the MGN. Even though the auditory pathway to the MGN has been cut, the retinal fibers do not invade the MGN, but they do so in EphrinA2, EphrinA5 double knockouts. © 2019 Elsevier Inc. All rights reserved. 8 Fig. 6.8 Regeneration of topographic specificity. (A) Langley’s classic study showed that stimulation of preganglionic root T4 relayed through ganglion cells in the SCG that caused vasoconstriction of the ear pinna vessels, whereas stimulation of root T1 excited other SCG neurons that caused dilation of the pupil. (B) When Langley cut the sympathetic tract above T1, all these sympathetic reflexes were abolished, but with time they recovered. (C) Shows the specificity associated with this regeneration, such that the axons that enter the chain at T4 reinnervate the SCG cells that cause ear vasoconstriction, and the axons that enter at T1 reinnervate the cells that cause pupil dilation. © 2019 Elsevier Inc. All rights reserved. 9 Fig. 6.9 Topographic input into the sympathetic chain. (A) Electrophysiological studies show that the SCG receives input from many roots but primarily the more anterior ones. The ganglion at T5 receives its primary inputs from more posterior roots. (B) When the SCG is removed and replaced with another SCG, the axons that reinnervate it tend to be from more anterior roots. (C) When a T5 ganglion is put in place of the SCG, its neurons still tend to get innervated by more posterior roots even though the ganglion is in an anterior position. (D) This topographic specificity of reinnervation is reflected in the shape of the histogram of EpSp amplitudes as a function of nerve root stimulation for the homototopic and heterotopic transplants. © 2019 Elsevier Inc. All rights reserved. 10 Fig. 6.10 Maladaptive topography implies chemospecificity. (A) Retinal ganglion cell axons map topographically onto the tectum in fish and amphibians, and regenerate following nerve crush to reinnervate their original topographic locations. (B) A normal frog sees a fly above on its ventral retina, which projects to the dorsal tectum, leading to a snap in the appropriate upward direction. (C) A frog with a rotated eye sees the same fly on what used to be the dorsal retina, which projects as ever to the ventral tectum, leading to a snap in the wrong downward direction. © 2019 Elsevier Inc. All rights reserved. 11 Fig. 6.11 The striped carpet assay. (A) An equatorial strip of retina spanning the nasal (N) temporal (T) extent is positioned on a striped carpet of alternating anterior (A) and posterior (P) tectal membranes. The nasal fibers from the retinal explant grow on both A and P tectal membranes, but the temporal fibers grow only on the A membranes. (B) If the tectal membranes are denatured or treated with PI-PLC, which releases PI-linked membrane proteins, the temporal axons also grow on both types of membranes, suggesting that the P membranes normally have a PI-linked repulsive guidance molecule. © 2019 Elsevier Inc. All rights reserved. 12 Fig. 6.12 Nasotemporal to anteroposterior retinotopic guidance system. (A) There is a gradient of Ephrins in the tectum, high in the posterior or caudal (C) pole and low in the anterior or rostral (R) pole. (B) A retinal ganglion cell in the temporal retina expresses active receptors for these tectal Ephrins and avoids the posterior tectum. (C) and (D) The opposing gradients of active Eph-A receptor expressed in the retina and the gradients of A-type Ephrins in the tectum. This system can at least partially account for topographic mapping in this axis. (E) The Ephrin-A gradient is shown in the tectum of a chick that uses a soluble Eph-A receptor fused to alkaline phosphatase to reveal the distribution of the Ephrin-A ligands in the tectum. © 2019 Elsevier Inc. All rights reserved. 13 Fig. 6.13 Topographic mapping in Ephrin-A2 and Ephrin-A5 double knockouts and with mosaic Eph-A3 misexpression. (A) Label is injected into the temporal (red, left) or nasal (green, right) retinas. (B) The result in the normal mice is a projection to the anterior (left) or posterior (right) colliculus. (C) In the Ephrin-A2, Ephrin-A5 double knockouts (tectum is gray), termination zones are all over the A-P extent of the colliculus for both nasal and temporal injections. (D) Two separate overlapping maps form from the subset of RGCs that express Isl2 and thus extra Eph-A3 (blue), and those that express the normal amount of Eph-A3 (red)—see graph below and micrograph of retinal axons from neighboring dye injection sites in the retina projecting to two distinct locations in the tectum, while maintaining their nearest neighbor topography. © 2019 Elsevier Inc. All rights reserved. 14 Fig. 6.14 Retinal axon outgrowth with variation in both retinal position and Ephrin-A2 concentration. Representative photographs showing outgrowth from the eight contiguous explant positions (numbered 1–8) across the nasal-temporal axis of the retina grown on substrates containing different proportions of membranes from Ephrin-A2 DNA-transfected and untransfected cells. Outgrowth varies with both retinal position and Ephrin concentration. Responses to Ephrin-A2 membranes vary from total outgrowth inhibition (at higher concentrations and more temporal positions) to severalfold outgrowth promotion (at lower concentrations and nasal positions). © 2019 Elsevier Inc. All rights reserved. 15 Fig. 6.15 Topographic mapping of the dorsal-ventral retina onto the mediolateral tectum.
Details
-
File Typepdf
-
Upload Time-
-
Content LanguagesEnglish
-
Upload UserAnonymous/Not logged-in
-
File Pages33 Page
-
File Size-