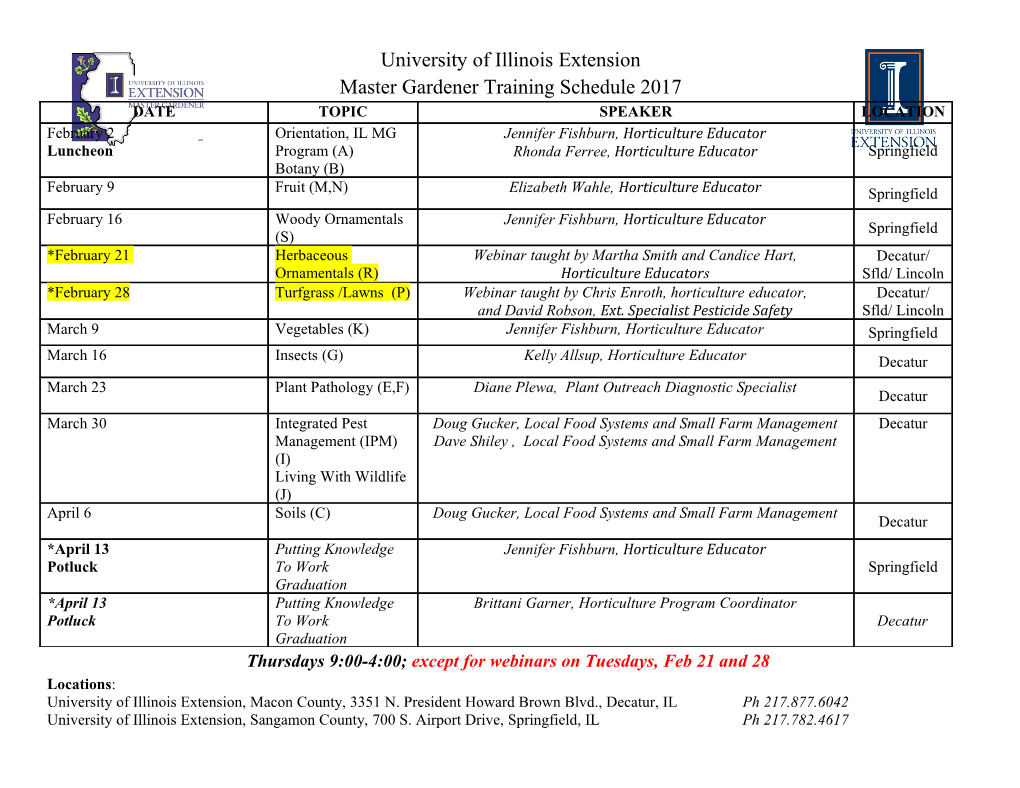
NANOIONIC PROTON CONDUCTIVITY ENHANCEMENT IN SOLID-STATE REACTIVE SINTERED BaCe0:7Zr0:1Y0:1Yb0:1O3−δ by Daniel Ryan Clark A thesis submitted to the Faculty and Board of Trustees of the Colorado School of Mines in partial fulfillment of the requirements for the degree of Master of Science (Materials Science). Golden, Colorado Date: Signed: Daniel Ryan Clark Signed: Dr. Ryan P. O’Hayre Associate Professor Thesis Advisor Golden, Colorado Date: Signed: Dr. Michael J. Kaufman Professor and Head Department of Metallurgical and Materials Engineering ii ABSTRACT The "holy grail" in the field of proton-conducting oxides is to decrease their minimum oper- ating temperature so that they may be used in a wider variety of applications. In addition to this challenge, new synthesis techniques are needed so that these materials can be produced with greater ease and lower cost. The possibility of exploiting nano-scale interfacial phenomena in solid-state ionic materials has risen to prominence. However, this approach, dubbed "nanoionics" by the scien- tific community, has not been widely applied to proton conductors, with only a few studies showing modest improvements. In this study, a novel approach is taken to create nanoionic interfaces in a high-performing proton conductor, BaCe0:7Zr0:1Y0:1Yb0:1O3−δ (BCZYYb). Thin nickel metal films are created at the grain boundaries of the BCZYYb bulk phase, creating space-charge layers at the interfaces. These nanoionic space-charge layers create a dramatic enhancement (up to 32X) in ionic conductivity. Using isotope and concentration cell experiments, which have never before been conducted on a proton conductor/metal nano-composite, it is shown that the enhancement is indeed protonic, suggesting space-charge layer enhancement. In addition to demonstrating a large nanoionic enhancement, the synthesis technique employed in this work used offers commercial viability. Using the recently discovered solid-state reactive sintering (SSRS) technique, these membranes are 1/10th the cost of normal polymeric/sol-gel synthesized materials, and in addition require less processing time due to the removal of the calcination step and the lower required sintering temperatures. iii TABLE OF CONTENTS ABSTRACT ::::::::::::::::::::::::::::::::::::::::::::: iii LIST OF FIGURES ::::::::::::::::::::::::::::::::::::::::: vi LIST OF TABLES :::::::::::::::::::::::::::::::::::::::::: ix ACKNOWLEDGMENTS :::::::::::::::::::::::::::::::::::::: x CHAPTER 1 INTRODUCTION ::::::::::::::::::::::::::::::::: 1 1.1 Motivation . .1 1.2 Applications . .2 1.3 Thesis Organization . .3 CHAPTER 2 BACKGROUND :::::::::::::::::::::::::::::::::: 4 2.1 Proton Conducting Oxides . .4 2.1.1 Barium Cerates . .5 2.1.2 Barium Zirconates . .6 2.1.3 Barium Cerate-Zirconates . .7 2.2 Solid-State Reactive Sintering . 10 2.3 Nanoionics and the Space-Charge Region . 13 2.3.1 Homogeneous Systems . 14 2.3.2 Heterogeneous Systems . 18 CHAPTER 3 EXPERIMENTAL RESULTS AND DISCUSSION ::::::::::::::: 27 3.1 Experimental Design . 27 3.1.1 Synthesis and Fabrication . 28 3.1.1.1 Solid-State Reactive Sintering . 28 3.1.1.2 Polymeric Synthesis . 28 3.1.1.3 Nickel Reduction . 30 3.1.2 X-ray Diffraction . 30 3.1.3 Electron Microscopy . 30 iv 3.1.3.1 FESEM . 30 3.1.3.2 FIB . 30 3.1.3.3 TEM . 31 3.1.4 Electrochemical Characterization . 31 3.1.4.1 Electrochemical Impedance Spectroscopy . 31 3.1.4.2 Hydrogen/Deuterium Isotope Experiment . 32 3.1.4.3 Concentration Cell . 33 3.2 Results and Discussion . 36 3.2.1 X-ray Diffraction . 37 3.2.2 Electron Microscopy . 37 3.2.3 Magnetometry . 41 3.2.4 Electrochemical Impedance Spectroscopy . 43 3.2.5 Hydrogen/Deuterium Isotope Experiment . 46 3.2.6 Concentration Cell . 47 3.2.7 Discussion . 49 CHAPTER 4 CONCLUDING REMARKS ::::::::::::::::::::::::::: 51 4.1 Summary and Conclusions . 51 4.2 Future Work . 51 REFERENCES CITED ::::::::::::::::::::::::::::::::::::::: 53 v LIST OF FIGURES 2.1 Cubic perovskite crystal structure, ABX3...........................5 2.2 Nyquist plot (imaginary vs. real impedance) of BZY10 and BCY10 single crystals. .7 ◦ 2.3 Conductivities of acceptor-doped compositions for the BaCeO3-BaZrO3 system at 100 C and 600 ◦C. ...........................................8 2.4 Conductivities of BaCe0:7Zr0:1Y0:2−xYbxO3−δ where 0:0 ≤ x ≤ 0:2 at different tempera- tures (◦C).............................................9 2.5 (a) Ionic conductivities of BCZYYb, BCZY, GDC, and YSZ from 400 to 700 ◦C in wet oxygen (∼3 vol. % H2O). (b) Typical current-voltage behavior for Ni-BCZYYb | BCZYYb | BCZY-LSCF and Ni-BCZYYb | SDC | LSCF in H2,H2 with H2S and dry propane at 750 ◦C. ....................................... 10 2.6 Secondary electron SEM images of fracture cross-sections of BZY20 with different sin- tering aids (∼1 wt. %) sintered at 1500 ◦C for 24 hours. 12 2.7 Secondary electron SEM images of fracture cross-sections of BCY20 with different amounts of NiO sintered at 1500 ◦C for 24 hours. 13 2.8 Examples of a heterogeneous interface with Phase 1 having a higher work function than Phase 2 (Φ1 > Φ2), giving rise to a space-charge layer. 14 2.9 Percentage of atoms in grain boundaries as a function of grain size assuming grain bound- ary widths of 0.5 and 1 nm. 15 •• 2.10 Oxygen vacancy (VO ) profiles of 8YSZ and 8YZA (8YSZ + 0.4 mol.% Al2O3) in the space-charge region. 17 2.11 HRTEM image of nanocrystalline ceria and conductivity as a function of pO2....... 17 2.12 Comparison of the electrical conductivity of SrCe0:95Yb0:05O3 (a) thin film on Al2O3 substrate (dg = 70 nm) and (b) polycrystal (dg = 3 µm) as a function of hydrogen con- centration at 1000 ◦C. ..................................... 19 ◦ 2.13 Conductivity of LiI/Al2O3 electrolyte as a function of Al2O3 content at 25 C. 20 2.14 Conductivity enhancement of AgX (X = Br,I) systems using mesoporous alumina parti- cles (MPA) at 25 ◦C....................................... 21 2.15 (a) Conductivity of CaF2/BaF2 heterolayers with interfacial densities in the 430 - 16 nm range. (b) The concentration or (parallel) conductivity profiles for the semi-infinite space- charge situation (left) and for the mesoscale situation (right) in which the space share regions overlap and the bulk values are exceeded even in the centers of the individual layers. .............................................. 22 vi 2.16 Conductivity of STO|YSZ|STO trilayers as a function of YSZ layer thickness and a high resolution dark-field STEM micrograph of the interfacial region and a comparison to thin film and single crystal YSZ. 23 ◦ 2.17 TEM bright field micrographs of highly reduced (dry 5% H2 for 24 hours at 650 C) BZY15Pd2.0 and Pd nanoparticles. 24 2.18 Backscatter electron images (composition) of polished cross-section of as-sintered BZY15 ◦ control, as-sintered BZY15Pd2.0, and highly reduced (dry 5% H2 for 24 hours at 650 C) BZY15Pd2.0 and the corresponding Pd-L and Y-L EDS maps. 25 2.19 Grain boundary, bulk, and total conductivity versus temperature for BZY20Pd2.0 com- pared with sol-gel control BZY15 (BZY15 Control) for highly reducing (dry 5% H2 for 24 ◦ ◦ hours at 650 C) and moderately reducing (wet 5% H2 for 24 hours at 650 C) conditions. 26 3.1 Block diagram comparing traditional polymeric precursor synthesis routes against solid- state reactive sintering for yttrium-doped barium zirconate. 29 3.2 Nyquist plot for BCZYYbNiO1 in a wet (pH2O ∼ 0.03 atm) 5% H2 (bal. Ar) environment at 100 ◦C and the equivalent circuit model used to solve for the bulk and grain boundary resistances. 32 3.3 Schematic of concentration cell reactor design. Outside tube is made of quartz and the inside tubes are made of alumina. 36 3.4 Schematic of concentration cell experiment gas flow. 37 3.5 X-ray diffraction comparison (CuKα) between BCZYYbNiO1 and BCZYYb-C from 10 - 120◦ 2θ. Peak intensities have been normalized for comparison. 38 3.6 Secondary electron images of fractured cross-sections of BCZYYb-C (sintered at 1450 ◦C for 24 h), BCZYYbNiO1 (before reduction - 1350 ◦C for 24 h) and a solid-state BCZYYb (1500 ◦C for 24 h) which had no NiO added to the oxide precursors. 39 3.7 Electron back-scatter image (compositional) of a polished cross section of BCZYYbNiO1 (sintered at 1350 ◦C for 24 h) before reduction and the corresponding EDS maps for BaL, CeL, NiK, YL, YbM, and ZrL x-rays. 40 3.8 Electron back-scatter image (compositional) of a polished cross section of BCZYYbNiO1 ◦ ◦ (sintered at 1350 C for 24 h) after reduction (5% H2 bal. Ar for 48 h at 750 C) and the corresponding EDS maps for BaL, CeL, NiK, YL, YbM, and ZrL x-rays. 40 3.9 Bright-field TEM images of BCZYYbNiO1 (sintered at 1350 ◦C for 24 h) after reduction ◦ (5% H2 bal. Ar for 48 h at 750 C)............................... 41 3.10 EDS spectrum from 0 - 20 keV of BCZYYbNiO1 (sintered at 1350 ◦C for 24 h) after ◦ reduction (5% H2 bal. Ar for 48 h at 750 C) for a grain boundary with Ni compared to the bulk. TEM micrograph of the measured region is shown. Unlabeled peak at 8.04 keV corresponds to CuKα which is caused by the Cu grid. 42 3.11 Schematic demonstrating the calculation of Ni metal concentration by subtracting the diamagnetic response (χdia) from the total M vs. H signal. 43 3.12 SQUID magnetometry data for BCZYYbNiO1 at 50 K for as-sintered, reduced (5% H2 ◦ bal. Ar for 48 h at 750 C), and reduced conductivity (measured in 5% H2 bal. Ar after reduction for ∼400 h). ..................................... 44 vii 3.13 SQUID magnetometry data for BCZYYbNiO1 at 150 K for as-sintered, reduced (5% ◦ H2 bal. Ar for 48 h at 750 C), and reduced conductivity (measured in 5% H2 bal. Ar after reduction for ∼400 h) This is the data set that was used to calculate Ni metal concentrations.
Details
-
File Typepdf
-
Upload Time-
-
Content LanguagesEnglish
-
Upload UserAnonymous/Not logged-in
-
File Pages74 Page
-
File Size-