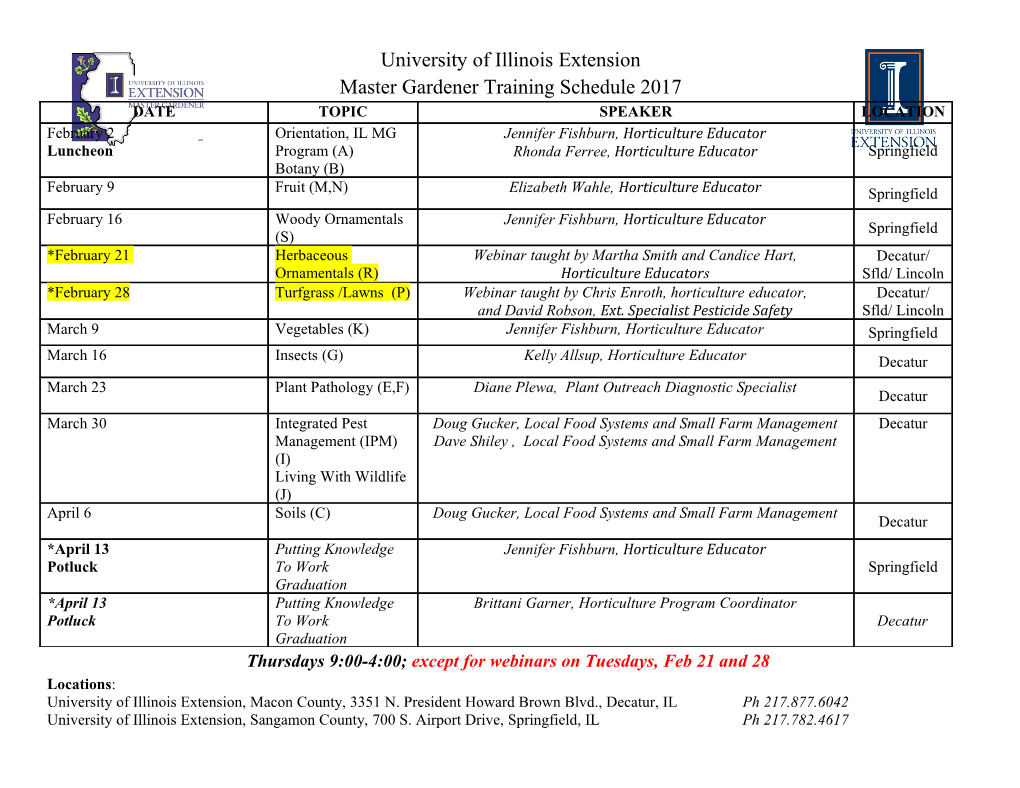
Impact of Nitrogen Profile in Gate Nitrided-Oxide on Deep-Submicron CMOS Performance and Reliability v Kanetake Takasaki v Kiyoshi Irino v Takayuki Aoyama v Youichi Momiyama v Toshiro Nakanishi v Yasuyuki Tamura v Takashi Ito (Manuscript received December 25, 2002) Silicon dioxide has been used for the gate insulator in CMOS with gate lengths down to 0.25 µm. However, when we enter the sub-0.18 µm era, nitrogen atoms must be incorporated into the silicon dioxide to prevent an undesirable penetration of boron atoms from the gate electrode to the Si substrate. In this paper, we describe the ef- fects of the nitrogen atom profile on CMOS performance and reliability and clarify the mechanisms underlying these effects. We show that high-performance, high-reliability CMOSFETs can be achieved by using a newly developed nitrided-oxide process that features a 900°C gate nitrided-oxide and establishes different nitrogen concentrations between the gate and extension area. When we enter the sub-100 nm-gate-length era of CMOS, we will need to replace thermal nitridation for the gate oxide with an alterna- tive nitridation process, for example, plasma nitridation. 1. Introduction current drivability due to depletion of the poly- Without an exquisite combination of silicon- crystalline-silicon electrode.5) dioxide (SiO2) and Si, we could not have enjoyed Fujitsu has a long history of comprehensive- the benefits of IT (Information Technology) tech- ly studying nitrided-oxide gate dielectrics, 6),7) and nologies based on state-of-the-art CMOS to address these issues caused by boron diffusion, (Complementary Metal-Oxide-Semiconductor) we have tried to replace gate SiO2 with nitrided- technology. oxide. Nitrided-oxide gate dielectrics (Gate-NO) As long as CMOS gate lengths stay around have been used for dual-gate CMOSFETs with 0.25 µm, we do not need to worry about using deep submicron channel lengths because of their silicon dioxide as a gate-insulator. However, when high boron blocking ability8),9) (Figure 1) and hot we enter the sub-0.18 µm-gate-length era of carrier immunity.10),11) However, the most serious CMOS, for the first time we face the need to re- problem with Gate-NO is that it deteriorates p- 12) place SiO2 with another gate insulator. To achieve MOSFET drivability. Preventing extension a high-performance, 0.18 µm-gate-length surface- dopants, especially boron, from diffusing outward channel p-MOSFET, we need to use into the sidewall and increasing the parasitic se- p+-poly-crystalline-silicon for the gate material.1) ries resistance (Rext) is expected to be another However, boron penetration from the p+-poly- important issue. To address these issues, we in- crystalline-silicon gate into the underlying silicon serted a nitrided-oxide (Ex-NO) between the substrate degrades device operation. This degra- extension area and sidewall as a blocking layer dation includes instability in the threshold voltage (Figure 2). We devised a novel way to indepen- (Vth), an increase in the charge-trapping rate, a dently optimize the nitrogen profile and content decrease in low-field mobility,2)-4) and a reduced for the Gate-NO and Ex-NO to suppress the deg- 40 FUJITSU Sci. Tech. J., 39,1,p.40-51(June 2003) K. Takasaki et al.: Impact of Nitrogen Profile in Gate Nitrided-Oxide on Deep-Submicron CMOS Performance and Reliability Annealing temperature (°C) <Pure oxide> <Nitrided oxide> 1200 1100 1000 900 800 Gate Gate -15 B Gate-NO 10 B B /s) -2 B B 2 + B Ex-NO BF2 : 5E15 cm B B B -16 Oxynitride B B 10 B B B B B B B B B B B B Nitrogen -17 Extension 10 B layer 0% Rext(PO) Rchannel Rext(NO) Rchannel 10-18 > Rext(PO) Rext(NO) 10-19 4% 25% 18% 9% Boron diffusion coefficient (cm Figure 2 10-20 0.65 0.7 0.75 0.8 0.85 0.9 0.95 Schematic cross section of p-MOSFET described in this paper. 1000/T (K-1) Figure 1 Boron diffusion coefficient in oxynitride. Furnace Process Quartz Temperature Nitridation O , N NO, N 2 2 2 tube 900°C Oxidation 750°C H2, N2 300°C Purge (Steam) (N2 gas) (NO gas) Pyrogenic Exhaust reactor NO O N SiO2 Si3N4 Si Si Figure 3 Gate nitrided-oxide process. radation of p-MOSFET drivability and the out- same furnace after gate electrode formation and ward diffusion of extension dopant. residual gate oxide removal. The process flow of the MOSFET is shown in Figure 4. After isola- 2. Experiments tion, a thin (3.5 to 5.5 nm) gate dielectric was The gate insulator formation furnace and grown. The gate dielectric thickness was deter- process are shown in Figure 3. As a control, gate mined by C-V measurement. Dual gate doping oxide was thermally grown in the furnace at 750 was carried out by B + and P + implantation after to 800°C. Gate-NO was grown by annealing ther- poly-Si deposition (180 nm). Shallow extension ° mal oxide in NO or N2O gas ambient at 800 C or was performed by low-energy implantation after ° 900 C. Ex-NO was also formed with NO in the Ex-NO formation. Sidewall formation (CVD-SiO2) FUJITSU Sci. Tech. J., 39,1,(June 2003) 41 K. Takasaki et al.: Impact of Nitrogen Profile in Gate Nitrided-Oxide on Deep-Submicron CMOS Performance and Reliability was followed by deep S/D implantation and RTA 3. Results (Rapid Thermal Annealing) at 1000°C. 3.1 Nitrided-oxide film properties We examined the nitrogen depth profile us- We examined the nitrogen depth profile by ing SIMS (Secondary Ion Mass Spectroscopy), the SIMS for three kinds of nitrided-oxide: 900°C-NO, nitrogen chemical bonding state using XPS (X-ray 800°C-NO, and 900°C-N2O (Figure 5). We also Photo-emission Spectroscopy), and the interface studied the nitrogen chemical bonding state us- property using charge pumping current measure- ing XPS. Figure 6 shows N1s spectra at various ment. We evaluated the characteristics of 0.2 µm take-off angles for the 900°C-NO and 800°C-NO. CMOSFETs and extracted the mobility and series The 900°C-NO has a sharp peak at about 398 eV, 13) ≡ resistance using the shift and ratio method. We which corresponds to the Si3 N bond in 14) ° also evaluated the hot carrier immunity for n- CVD-Si3N4. On the other hand, the 800 C-NO MOSFETs. has two peaks: one at about 398 eV and another at about 399 eV. The intensity of the 398 eV peaks increases with the take-off angle, and the intensity of the 399 eV peak decreases with the take-off angle. Gate-NO This indicates that the 398 and 399 eV peaks cor- After gate-NO respond, respectively, to a nitrogen-related bond STI near the interface and a nitrogen-related bond in the bulk nitrided-oxide.15) As the thickness of the Poly Si Ex-NO 800°C-NO is increased, the binding energy (BE) After extension-NO formation around 399 eV increases, but the BE around 398 eV remains almost constant (Figure 7). This CVD-SiO CoSi2 2 energy shift might be caused by the X-ray induced 16) After source drain charge-up effect, implying that the 399 eV peak formation ≡ also corresponds to Si3 N bonds and some nitro- gen atoms exist away from the interface. These Figure 4 results suggest that nitrogen atoms exist both at Process flow of MOSFET. the interface and in the bulk in the 800°C-NO 3 900°C-N O 2 Si ≡ N: 398 eV 800°C-NO 3 2.5 Gate 900°C-NO 900°C-NO 800°C-NO insulator 2 Si-substrate 1.5 Si profile ° ° 1 (arb. unit) 90 90 Nitrogen (at. %) Nitrogen (at. Intensity (a.u.) Intensity 0.5 ° (a.u.) Intensity 45 45° 20° 0 20° -4 -20 2 4 Depth (nm) 404 402 400 398 396 394 404 402 400 398 396 394 Binding energy (eV) Binding energy (eV) Figure 5 Nitrogen depth profile for 900°C-NO, 800°C-NO, and Figure 6 ° 900 C-N2O measured by secondary ion mass spectros- N1s photoelectron spectra for various take-off angles of copy. 900°C-NO and 800°C-NO by XPS. 42 FUJITSU Sci. Tech. J., 39,1,(June 2003) K. Takasaki et al.: Impact of Nitrogen Profile in Gate Nitrided-Oxide on Deep-Submicron CMOS Performance and Reliability 400 400 ° ° ° 900 C-N2O 800 C-NO 900 C-NO 900°C-NO 800°C-NO SiO2 SiO2 SiO2 Nitrogen 399 399 Interface Charge-up effect 398 398 Si Si Si binding energy (eV) binding energy (eV) s 1s 1 N N Mainly in Mainly at At interface bulk interface 397 397 2 2.5 3 3.5 4 4.5 5 2 2.5 3 3.5 4 4.5 5 Figure 8 Thickness (nm) Thickness (nm) Schematic cross section of nitrided oxide showing the lo- cation of nitrogen. Figure 7 Dependence of N1s binding energy on oxynitride ° ° thickness for 900 C-NO and 800 C-NO by XPS. ≡ ≡ ≡ (a) N Si3 (a’) N Si2O (b) N Si3 samples, but only at the interface in the 900°C-NO) samples.17) The XPS spectra for the ° 900 C-N2O mainly consisted of a 399 eV peak, showing that nitrogen atoms are located in the bulk (Figure 8).18) ≡ ≡ (c) N Si3 (d) N O3 NO A theoretical analysis based on first- Si principle calculation also confirms that the Relative energy ≡ Si3 N bonds at the interface are the most stable a 0.0 (eV) a' 2.4 and higher temperature thermal treatments in- b 0.7 duce nitrogen atoms to pile up at the SiO2/Si c 1.8 d 7.7 interface (Figure 9).19) 3.2 Nitrided-oxide interface properties Figure 9 ≡ Three-coordinated-N-configurations.
Details
-
File Typepdf
-
Upload Time-
-
Content LanguagesEnglish
-
Upload UserAnonymous/Not logged-in
-
File Pages12 Page
-
File Size-