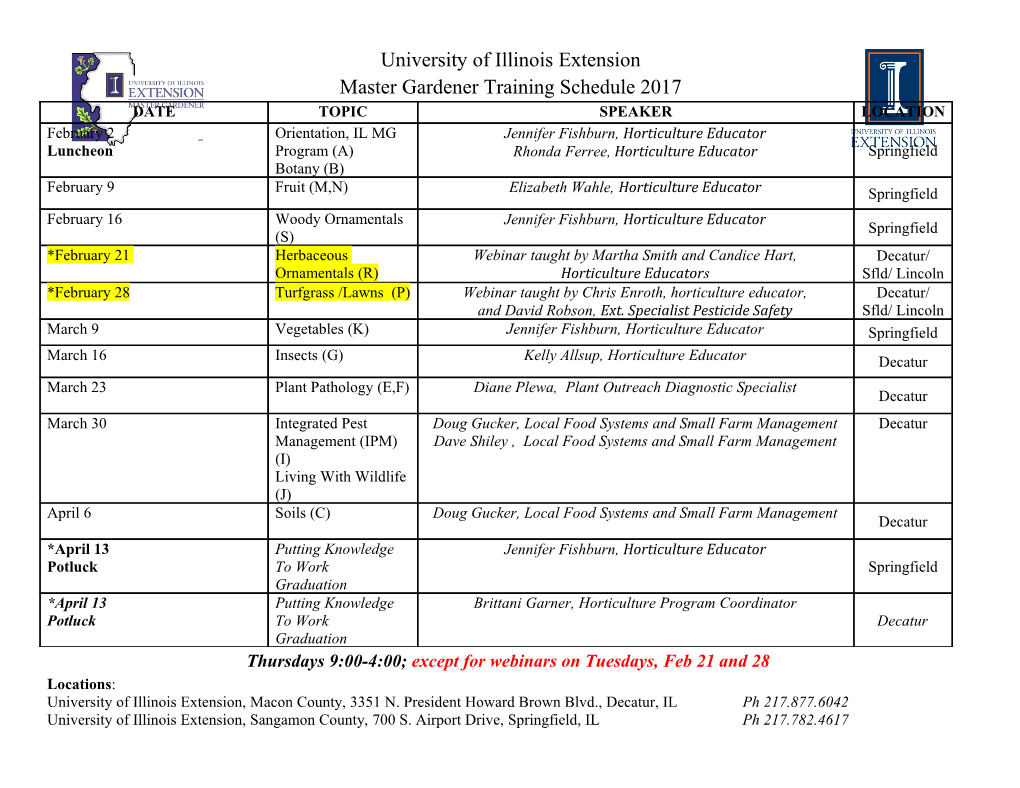
Measurement of the Cosmic Microwave Background Polarization with the Bicep Telescope at the South Pole by Yuki David Takahashi A dissertation submitted in partial satisfaction of the requirements for the degree of Doctor of Philosophy in Physics in the Graduate Division of the University of California, Berkeley Committee in charge: Professor William L. Holzapfel, Chair Professor Adrian T. Lee Professor Chung-Pei Ma Fall 2010 Measurement of the Cosmic Microwave Background Polarization with the Bicep Telescope at the South Pole Copyright 2010 by Yuki David Takahashi 1 Abstract Measurement of the Cosmic Microwave Background Polarization with the Bicep Telescope at the South Pole by Yuki David Takahashi Doctor of Philosophy in Physics University of California, Berkeley Professor William L. Holzapfel, Chair The question of how exactly the universe began is the motivation for this work. Based on the discoveries of the cosmic expansion and of the cosmic microwave background (CMB) ra- diation, humans have learned of the Big Bang origin of the universe. However, what exactly happened in the first moments of the Big Bang? A scenario of initial exponential expansion called “inflation” was proposed in the 1980s, explaining several important mysteries about the universe. Inflation would have generated gravitational waves that would have left a unique imprint in the polarization of the CMB. To search for this evidence for inflation, a team gathered in 2002 to design a telescope experiment called Bicep. Sited at the South Pole, Bicep was a novel 25-cm aperture refractor with 49 pairs of polarization-sensitive bolometers. We completed 3 years of successful observations from February 2006 to De- cember 2008. To constrain the amplitude of polarization resulting from inflation, expected to be at least 7 orders of magnitude fainter than the 3 K CMB intensity, precise control of systematic effects is essential. A crucial challenge is preventing systematic errors from introducing false polarization anisotropy signal at the level corresponding to ∼0.1 µK in amplitude. One main focus of this thesis is the characterization of systematic effects for Bi- cep. We developed a simulation framework for propagating instrumental systematic effects to the final polarization results. Based on these simulations, we established benchmarks for the characterization of critical instrumental properties including bolometer relative gains, beam mismatch, polarization orientation, telescope pointing, sidelobes, thermal stability, and timestream noise model. Guided by these benchmarks, we carefully measured these properties and have shown that we have characterized the instrument adequately to ensure that systematic errors do not limit Bicep's current cosmology results. We have analyzed the first 2 years of data, lowering the upper limits on the gravitational-wave induced polarization by an order of magnitude over all previous experiments. The systematic error analysis has identified what future refinements are likely necessary to probe CMB polarization down to levels corresponding to inflationary energy scales below 2 × 1016 GeV. i Contents List of Figures iii List of Tables v Acknowledgements vi 1 How did the universe begin? 1 1.1 Expanding universe . 2 1.2 Remnant radiation from the Big Bang . 5 1.3 Inflationary beginning of the Big Bang . 7 1.4 Evidence for inflation . 9 1.4.1 Inflationary gravitational waves . 9 1.4.2 Cosmic microwave background polarization . 9 1.4.3 Curl-mode polarization . 11 2 BICEP experiment concept 14 2.1 Design summary . 15 2.2 South Pole site . 16 2.3 Instrument . 18 2.3.1 Polarization sensitive bolometers . 18 2.3.2 Filters and feedhorns . 21 2.3.3 Cold HDPE lenses . 22 2.3.4 Infrared blockers . 24 2.3.5 Readout electronics . 24 2.4 Galactic foregrounds and sky coverage . 25 2.5 Observing strategy . 28 3 Hardware development 31 3.1 Vacuum window . 32 3.2 Ground screen and forebaffle . 34 3.3 Polarization calibrator . 37 3.4 Motion control system . 42 3.5 Optical star-pointing camera . 43 3.6 Refrigerator electronics . 45 3.7 Deployment . 50 ii 4 Instrument characterization 51 4.1 Calibration goals and systematic error simulation . 52 4.2 Relative detector gains . 54 4.2.1 Temporal transfer functions . 56 4.2.2 Relative responsivities . 58 4.2.3 Spectral response . 61 4.3 Beam characterization . 62 4.4 Polarization orientations and efficiencies . 65 4.5 Telescope and detector pointing . 68 4.6 Sidelobe rejection . 69 4.7 Thermal stability . 73 5 Data analysis and results 76 5.1 Collected data, cuts, and observing efficiency . 76 5.2 Data analysis overview . 78 5.3 CMB temperature and polarization maps . 78 5.4 Noise properties and modeling . 80 5.4.1 Properties of noise: spectra and covariance . 81 5.4.2 Simulation of noise-only timestreams . 82 5.4.3 Noise bias in power spectra . 85 5.5 Angular power spectra . 87 5.6 Conclusion . 89 Bibliography 90 iii List of Figures 1.1 Velocity-distance relation among extra-galactic nebulae . 2 1.2 Blackbody spectrum of the CMB measured by COBE .............. 6 1.3 Comoving scales and causal horizon during and after inflation . 8 1.4 Thomson scattering generating polarization . 10 1.5 Three perturbation types resulting in quadrupole anisotropy . 11 1.6 CMB angular power spectra. 12 2.1 Bicep telescope . 15 2.2 South Pole Dark Sector and LC-130 . 17 2.3 Polarization sensitive bolometer . 19 2.4 Layout of the Bicep beams . 20 2.5 Filters and feedhorns . 22 2.6 Optics tube housing two lenses . 23 2.7 Maps of expected levels of polarized foregrounds at 150 GHz . 26 2.8 Bicep's CMB and Galactic fields . 27 2.9 Azimuth scan profile used in mapping the CMB field . 29 2.10 48-hr observing cycle . 30 3.1 Diagram of window, forebaffle, calibrator, and optical camera . 31 3.2 Vacuum window and window cover . 32 3.3 Snow on the window cover sublimating . 33 3.4 Predicted beam patterns out of the aperture . 34 3.5 Grounds shields . 35 3.6 Photograph of absorptive forebaffle and reflective ground screen. 36 3.7 Dielectric sheet calibrator diagram . 38 3.8 Mylar sheet reflectivity for various thicknesses . 38 3.9 Dielectric sheet calibrator expected signal . 40 3.10 Dielectric sheet calibrator for measuring PSB orientations . 41 3.11 3-axis telescope mount, interlock system, and manual controller . 43 3.12 Motion control interlock scheme . 44 3.13 Optical star-pointing camera . 45 3.14 Adsorption refrigerator . 46 3.15 Electronics schematic for resistance thermometer readout . 47 3.16 Electronics schematic for heater drivers . 48 3.17 Electronics schematic for LHe level sensor readout . 49 3.18 Refrigerator electronics . 49 iv 4.1 Spurious BB power from simulations of measured potential systematic errors . 55 4.2 Temporal response function measurement setup . 57 4.3 Measured transfer functions . 58 4.4 Bolometer timestream during relative responsivity calibration . 59 4.5 Relative responsivity measurement consistency . 60 4.6 PSB pair-differencing . 61 4.7 Average measured spectral response . 62 4.8 Beam mapping setup on site . 63 4.9 Differential beam map . 64 4.10 Dielectric sheet calibrator response . 66 4.11 Setup above the cryostat window for measuring cross-polarization responses . 67 4.12 Calibration source on a mast for measuring cross-polarization responses . 67 4.13 Sidelobe measurement using broadband noise source on a mast . 69 4.14 Map of fractional polarization . 70 4.15 Azimuthally-averaged sidelobe response . 71 4.16 Integrated beam . 72 4.17 Convolution of sidelobes with Galactic emissions . 73 4.18 Power spectra of sidelobe response to Galactic emissions . 73 4.19 Focal plane thermistor map . 75 4.20 Power spectra of focal plane temperature fluctuations . 75 5.1 Bicep map of the entire mapped field . 77 5.2 Data analysis flow chart . 78 5.3 Maps from the first 2 years of data, including frequency jackknife differences . 79 5.4 Average noise frequency spectra of all PSB pairs . 82 5.5 Example correlation matrices from the Bicep noise model . 83 5.6 Noise model spectrum and the simulated timestream spectra . 84 5.7 Real and simulated-noise timestreams for a PSB pair . 85 5.8 Power spectra of simulated signal+noise, noise only, and data jackknife . 86 5.9 Bicep 2-year CMB polarization.
Details
-
File Typepdf
-
Upload Time-
-
Content LanguagesEnglish
-
Upload UserAnonymous/Not logged-in
-
File Pages105 Page
-
File Size-